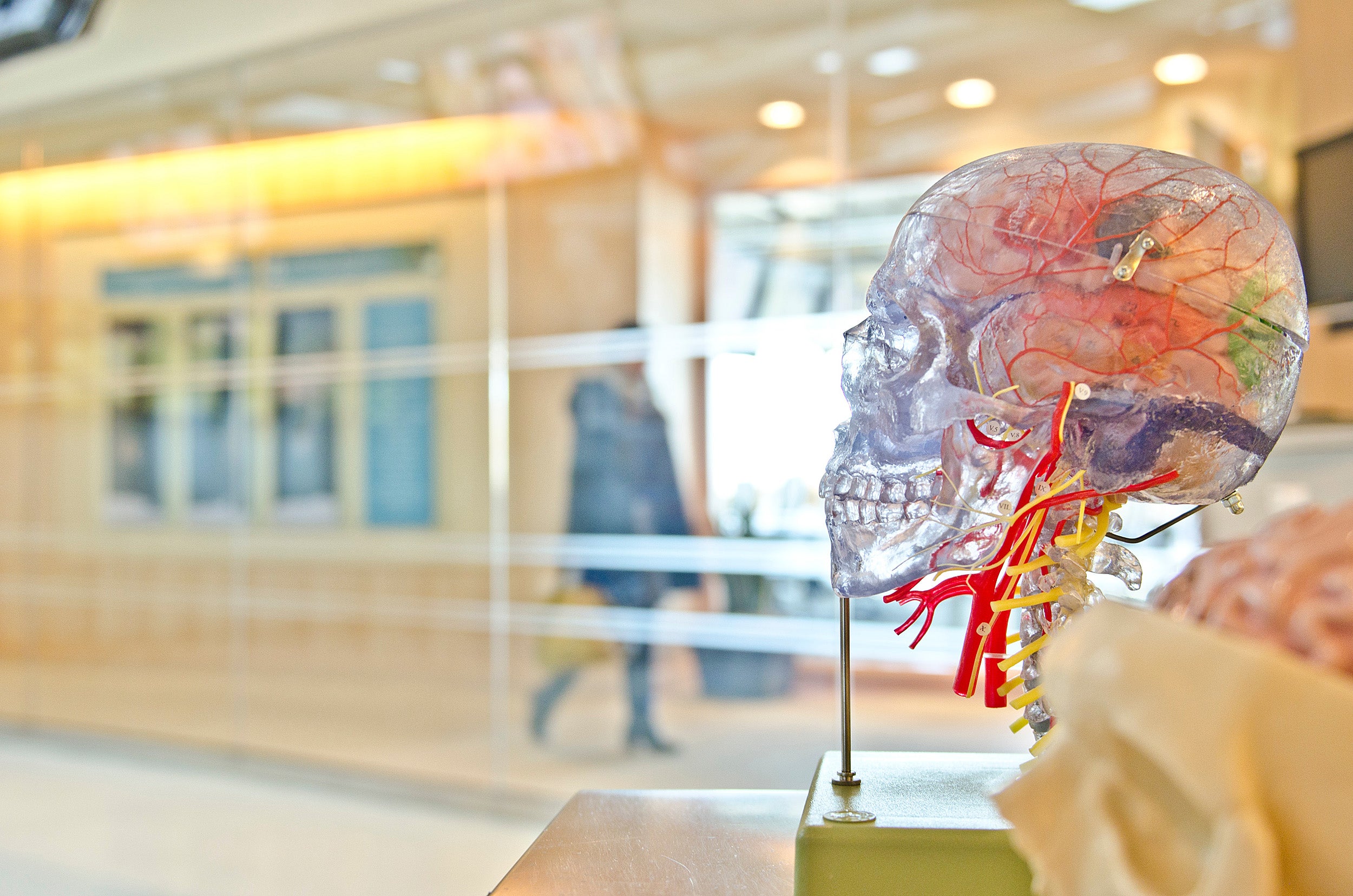
Wyss Institute scientists have developed chip technology that mimics the blood-brain barrier in humans. The new models will help researchers study drugs to treat cancer, neurodegeneration, and other diseases of the central nervous system.
Unsplash
Blood-brain barrier chip performs human-like drug and antibody transport
Model offers new tool for drug development
Like airport security barriers that either clear authorized travelers or block unauthorized ones from accessing central operation areas, the blood-brain barrier (BBB) tightly controls the transport of essential nutrients and energy metabolites into the brain and staves off unwanted substances circulating in the bloodstream. Its highly organized structure of thin blood vessels and supporting cells is also the major obstacle preventing lifesaving drugs from reaching the brain to effectively treat cancer, neurodegeneration, and other diseases of the central nervous system. In a number of brain diseases, the BBB can also break down locally, causing neurotoxic substances, blood cells, and pathogens to leak into the brain and wreak irreparable havoc.
To study the BBB and drug transport across it, researchers have mostly relied on animal models such as mice. However, the precise makeup and transport functions of BBBs in these models can significantly differ from those in human patients, which makes them unreliable for predicting drug delivery and therapeutic efficacies. Also, in vitromodels attempting to re-create the human BBB using primarily brain tissue-derived cells thus far have not been able to mimic the BBB’s physical barrier, transport functions, and drug and antibody shuttling activities closely enough to be useful as therapeutic development tools.
Now, a team led by Donald Ingber, founding director of Harvard’s Wyss Institute for Biologically Inspired Engineering, has overcome these limitations by leveraging its microfluidic organs-on-chips technology in combination with a developmentally inspired hypoxia-mimicking approach to differentiate human pluripotent stem (iPS) cells into brain microvascular endothelial cells (BMVECs). The resulting “hypoxia-enhanced BBB chip” recapitulates the cellular organization, tight barrier functions, and transport abilities of the human BBB, while allowing transport of drugs and therapeutic antibodies in a way that more closely mimics transport across the BBB in vivo than existing in vitro systems do. Their study is reported in Nature Communications.
“Our approach to modeling drug and antibody shuttling across the human BBB in vitro with such high and unprecedented fidelity presents a significant advance over existing capabilities in this enormously challenging research area,” said Ingber, who is also the Judah Folkman Professor of Vascular Biology at Harvard Medical School and professor of bioengineering at the John A. Paulson School of Engineering and Applied Sciences. “It addresses a critical need in drug-development programs throughout the pharma and biotech world that we now aim to help overcome with a dedicated brain-targeting program at the Wyss Institute using our unique talent and resources.”
The BBB consists of thin capillaries formed by BMVECs, multifunctional cells known as pericytes that wrap themselves around the outside of the vessels, and star-shaped astrocytes, which are non-neuronal brain cells that also contact blood vessels. In the presence of pericytes and astrocytes, endothelial cells can generate the tightly sealed vessel-wall barrier typical of the human BBB.
Ingber’s team first differentiated human iPS cells into brain endothelial cells in a culture dish using a method that was developed by co-author Eric Shusta, a professor of chemical and biological engineering at University of Wisconsin-Madison, but with the added power of bioinspiration. “Because in the embryo the BBB forms under low-oxygen conditions [hypoxia], we differentiated iPS cells for an extended time in an atmosphere with only 5 percent instead of the normal 20 percent oxygen concentration,” said co-first author Tae-Eun Park. “As a result, the iPS cells initiated a developmental program very similar to that in the embryo, producing BMVECs that exhibited higher functionality than BMVECs generated in normal oxygen conditions.” Park is a former postdoctoral fellow on Ingber’s team and is now an assistant professor at Ulsan National Institute of Science and Technology in the Republic of Korea.
Building on a previous human BBB model, the researchers next transferred the hypoxia-induced human BMVECs into one of two parallel channels of a microfluidic organ-on-chip device that are divided by a porous membrane and continuously perfused with medium. The other channel was populated with a mixture of primary human brain pericytes and astrocytes. Following an additional day of hypoxia treatment, the human BBB chip could be stably maintained for at least 14 days at normal oxygen concentrations, which is far longer than in vitro human BBB models attempted in the past.
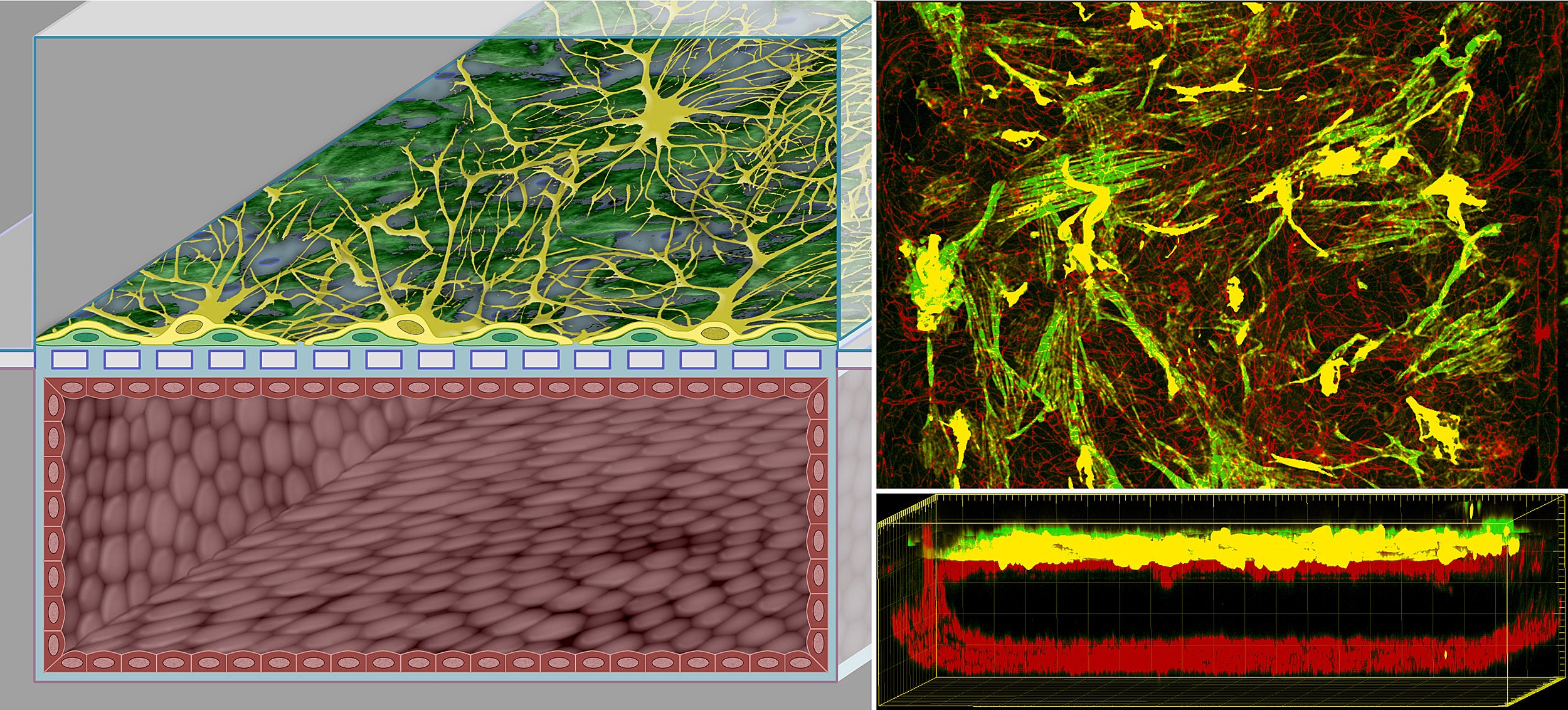
In the enhanced human blood brain barrier chip, cells derived from human stem cells form a microvessel in the lower of two parallel channels. At the same time, pericytes (spatially isolated cells on capillaries) and astrocytes (star-shaped cells in the brain) populate the upper channel and connect to the microvessel across a dividing porous membrane.
Wyss Institute at Harvard University
Under the shear stress of the fluids perfusing the BBB chip, the BMVECs go on to form a blood vessel and develop a dense interface with pericytes, aligning with them on the other side of the porous membrane, as well as with astrocytes extending toward them through small openings in the membrane. “The distinct morphology of the engineered BBB is paralleled by the formation of a tighter barrier containing elevated numbers of selective transport and drug shuttle systems compared to control BBBs that we generated without hypoxia or fluid shear stress, or with endothelium derived from adult brain instead of iPS cells,” said Nur Mustafaoglu, a co-first author on the study and a postdoctoral fellow working on Ingber’s team. “Moreover, we could emulate effects of treatment strategies in patients in the clinic. For example, we reversibly opened the BBB for a short time by increasing the concentration of a mannitol solute [osmolarity] to allow the passage of large drugs like the anti-cancer antibody cetuximab.”
To provide additional proof that the hypoxia-enhanced human BBB chip can be used as an effective tool for studying drug delivery to the brain, the team investigated a series of transport mechanisms that either prevent drugs from reaching their targets in the brain by pumping them back into the bloodstream (efflux), or allow the selective transport of nutrients and drugs across the BBB (transcytosis).
“When we specifically blocked the function of P-gp, a key endothelial efflux pump, we could substantially increase the transport of the anti-cancer drug doxorubicin from the vascular channel to the brain channel, very similarly to what has been observed in human patients,” said Park. “Thus, our in vitro system could be used to identify new approaches to reduce efflux and thus facilitate drug transport into the brain in the future.”
On another venue, drug developers are trying to harness “receptor-mediated transcytosis” as a vehicle for shuttling drug-loaded nanoparticles, larger chemical and protein drugs, and therapeutic antibodies across the BBB. “The hypoxia-enhanced human BBB chip recapitulates the function of critical transcytosis pathways, such as those used by the LRP-1 and transferrin receptors responsible for taking up vital lipoproteins and iron from circulating blood and releasing them into the brain on the other side of the BBB,” said Mustafaoglu. “By harnessing those receptors using different preclinical strategies, we can faithfully mimic the previously demonstrated shuttling of therapeutic antibodies that target transferrin receptors in vivo, while maintaining the BBB’s integrity in vitro.”
Based on these findings, the Wyss Institute has initiated a brain-targeting program. “Initially, the BBB transport program aims to discover new shuttle targets that are enriched on the BMVEC vascular surface, using novel transcriptomics, proteomics, and iPS cell approaches. In parallel, we are developing fully human antibody shuttles directed against known shuttle targets with enhanced brain-targeting capabilities,” said James Gorman, the staff lead on the BBB transport program’s advanced technology team. “We aim to collaborate with multiple biopharmaceutical partners in a pre-competitive relationship to develop shuttles offering exceptional efficacy and engineering flexibility for incorporation into antibody and protein drugs, because this is so badly needed by patients and the whole field.”
The authors think that in addition to drug development studies, the hypoxia-enhanced human BBB chip can also be used to model aspects of brain diseases likely affected by the breakdown of the BBB, such as Alzheimer’s and Parkinson’s disease, and how those diseases in turn affect the BBB, as well as to advance personalized medicine approaches by using patient-derived iPS cells.
The study was funded by Harvard’s Wyss Institute for Biologically Inspired Engineering, the Defense Advanced Research Projects Agency (DARPA), the National Research Foundation of Korea, and the Knut and Alice Wallenberg Foundation.