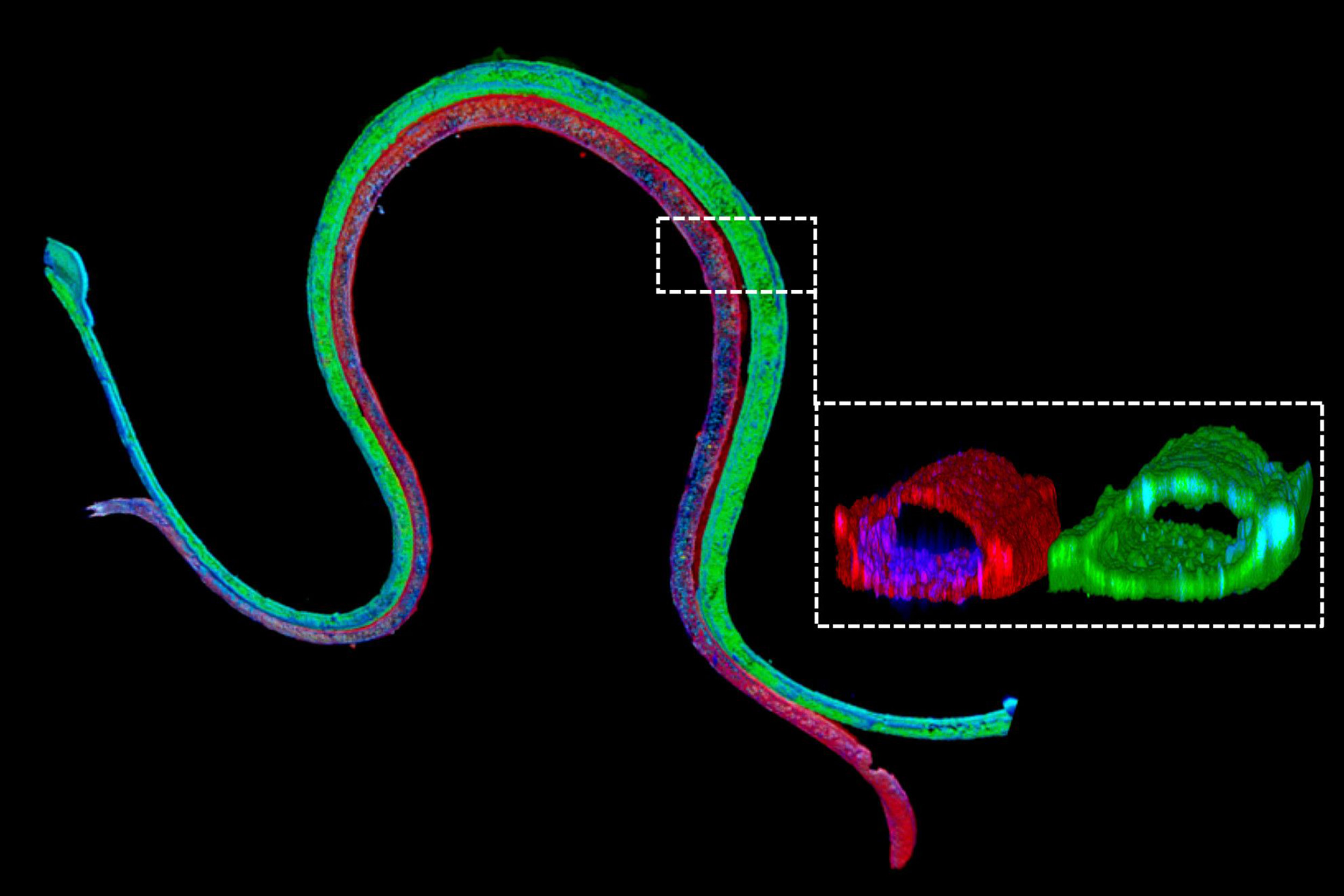
Immunofluorescence staining of a 3-D bioprinted tubule. The magnified cross-section illustrates that the two different cell types form luminal perfusable structures in their respective channels.
Wyss Institute at Harvard University
A step closer to tissue-engineered kidneys
3-D bioprinted, vascularized proximal tubules mimic the human organ’s reabsorption functions
Every day our kidneys tackle the daunting task of continuously cleaning our blood to prevent waste, salt, and excess fluid from building up inside our bodies. To achieve this, the kidneys’ approximately 1 million minute filtration units, called glomeruli, first remove both waste products and precious nutrients from the bloodstream. Then, specialized structures known as the proximal tubules reabsorb the “good” molecules — glucose, amino acids, some vitamins, and electrolytes — returning them to the bloodstream.
But the reabsorptive functions of the proximal tubules can be compromised by drugs, chemicals, and genetic and blood-borne diseases. Because our understanding of how these effects occur is still limited, researchers have been working to replicate proximal tubes and other kidney structures in the lab so they can better study their functions, screen drugs without testing on humans or animals, and ultimately use them as a foundation to engineer kidney replacements for diseased or damaged organs.
To help study renal reabsorption, in 2016 Wyss Institute core faculty member Jennifer Lewis and her team — working within the institute’s 3D Organ Engineering Initiative, which she co-leads, and in collaboration with the Roche Innovation Center Basel in Switzerland — created a 3-D proximal tubule model in which fluids could be continuously streamed through the tubules.
While that model removed molecules from the system, however, it lacked a functional blood-vessel compartment for picking up molecules again so they could be reabsorbed by the proximal tubules.
This week, Lewis’ team has presented a solution to that problem: a 3-D vascularized proximal tubule model they created in which independently perfusable tubules and blood vessels are printed adjacent to each other within an engineered extracellular matrix. Their study is published in the Proceedings of the National Academy of Sciences (PNAS).
“We construct these living renal devices in a few days and they can remain stable and functional for months,” said first author Neil Lin, a Roche Fellow and a postdoctoral fellow on Lewis’ team. These 3-D vascularized proximal tubules, Lin continued, demonstrate that the team’s multitissue constructs are indeed mature and functional. “[They] exhibit the desired epithelial and endothelial cell morphologies and luminal architectures, as well as the expression and correct localization of key structural and transport proteins and factors that allow the tubular and vascular compartments to communicate with each other,” he said.
As a first step toward testing drugs and modeling diseases, the team induced hyperglycemia, a high-glucose condition typical of diabetes and a known risk factor for vascular disease, into their model by circulating a fourfold-higher-than-normal glucose concentration through the proximal tubule compartment. “We found that high levels of glucose transported to endothelial cells in the vascular compartment caused cell damage,” said Kimberly Homan, a co-author of the study and a research associate in Lewis’ group at the Wyss Institute and the Harvard John A. Paulson School of Engineering and Applied Science (SEAS). “By circulating a drug through the tubule that specifically inhibits a major glucose transporter in proximal tubule epithelial cells, we prevented those harmful changes from happening to the endothelial cells in the adjacent vessels.”
The team’s immediate focus is to further scale up their new model for use in pharmaceutical applications. “Our system could enable the screening of focused drug libraries for renal toxicity and thus help reduce animal experiments,” said Annie Moisan, a co-author and industry collaborator on the study, and principal scientist at Roche Innovation Center Basel. “I am thrilled by the continued efforts from us and others to increase the physiological relevance of such models, for example by incorporating patient-specific and diseased cells, since personalized efficacy and safety are the ultimate goals of predicting clinical responses to drugs.”
“Our new 3-D kidney model is an exciting advance, as it more fully recapitulates the proximal tubule segments found in native kidney tissue,” said Lewis, who is also the Hansjörg Wyss Professor of Biologically Inspired Engineering at SEAS, the Jianmin Yu Professor of Arts and Sciences, and a member of the Harvard Stem Cell Institute. “Beyond its immediate applications for drug screening and disease modelling, we are also exploring whether these living devices can be used to augment kidney dialysis.” Currently, life-saving dialysis machines filter blood, but they are unable to retrieve from the filtrate the nutrients and other molecules that the body needs for many of its functions, which can cause specific deficiencies and complications down the line. Lewis and her colleagues believe that 3-D bioprinted vascularized tubules may lead to improved renal replacement therapies.
“This study presents a significant step forward in human kidney engineering that enables human disease and drug-related studies to be carried out over extended periods of time in vitro,” said Wyss Institute Founding Director Donald Ingber, who is also a professor of bioengineering at SEAS and the Judah Folkman Professor of Vascular Biology at HMS and the Vascular Biology Program at Boston Children’s Hospital. “It also represents a major step forward for the Wyss Institute’s 3D Organ Engineering Initiative, which aims to generate functional organ replacements with enhanced functionalities for patients in need.”
The study was also authored by present and past members of Lewis’ team Sanlin Robinson, David Kolesky, and Nathan Duarte. It was funded by grants from the National Institutes of Health, Harvard’s Wyss Institute for Biologically Inspired Engineering, a Roche Postdoctoral Fellowship, and a donation from the GETTYLAB.