Hinting at answer to a chicken-or-egg question on evolution
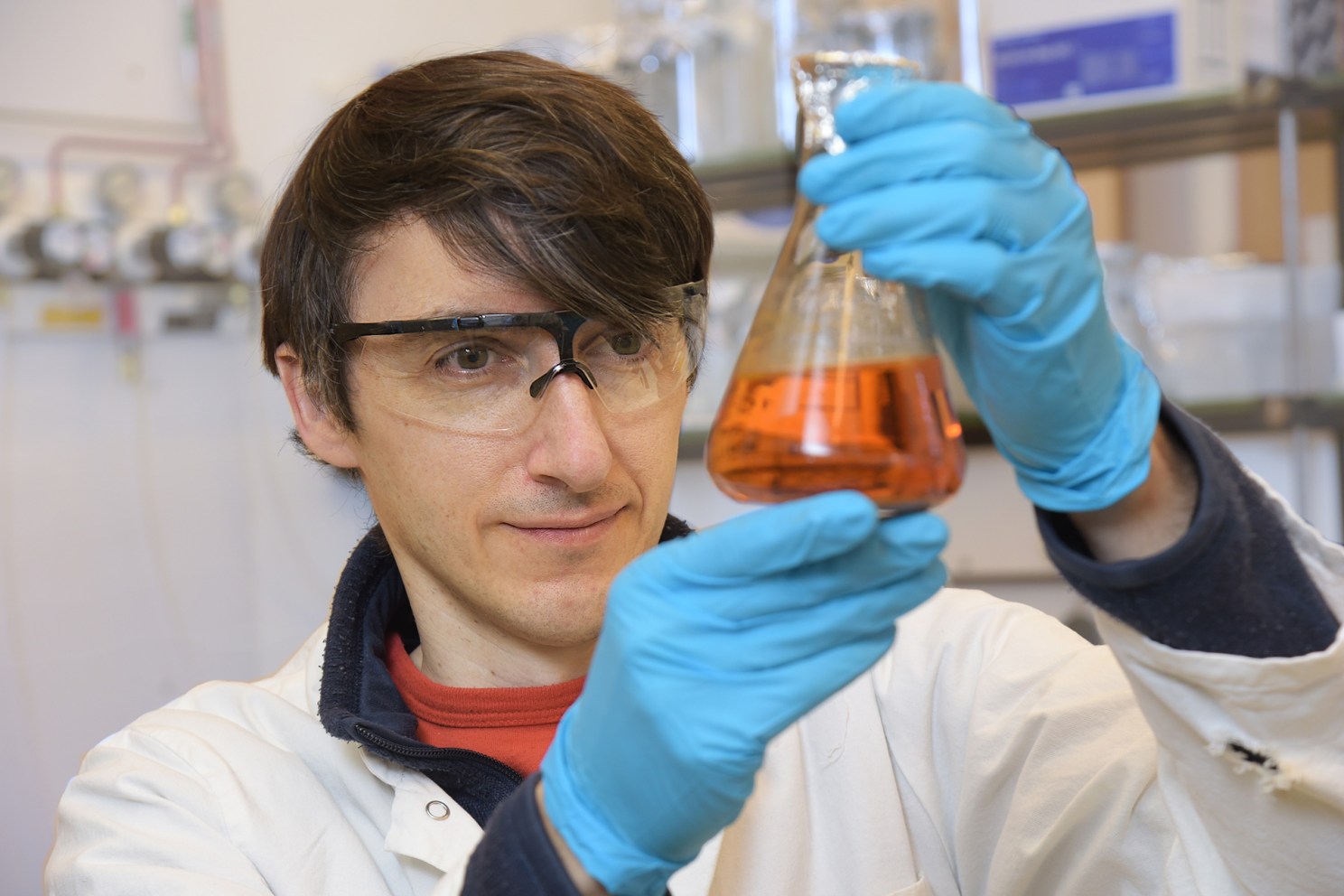
Felix Elling, a former postdoctoral fellow at Harvard and lead author of the study.
Courtesy of Felix Elling
Accidental find may help scientists resolve which evolved first: ability to produce oxygen by photosynthesis or consume it by aerobic metabolism
For biochemists, it’s the which-came-first question: oxygen production by photosynthesis or oxygen consumption by aerobic metabolism?
In photosynthesis, algae and plants take in sunlight to turn carbon dioxide and water into fuel for growth, releasing oxygen as a byproduct. Animals, on the other hand, use oxygen to convert the fuel they consume into energy and emit carbon dioxide, a process called aerobic metabolism.
So which came first? A new paper in the Proceedings of the National Academy of Sciences details an accidental discovery by an international consortium of researchers of a possible missing-link molecule that may lead to an answer to the evolutionary question.
“Right from the start we had this idea that this might be related to the evolution of photosynthesis and the ability to breathe oxygen,” said Felix Elling, a former postdoctoral fellow in the Department of Earth and Planetary Sciences and lead author on the paper.
Elling, who was working in Professor Ann Pearson’s Lab for Molecular Biogeochemistry and Organic Geochemistry, was looking for specific molecules unrelated to questions about the evolution of aerobic metabolism when he discovered something unusual: a slight change in a molecule in a nitrogen-utilizing bacterium, Nitrospirota, that appeared more like something that a plant would need for photosynthesis, rather than a bacterium.
“We were screening bacteria for a completely different project,” said Elling, who is now on the faculty at the University of Kiel in Germany.
What the researchers had found was methyl-plastoquinone, a variation on a molecule type called a quinone. Found in all life forms, quinones had been thought to exist in two basic varieties: aerobic quinones that require oxygen and anaerobic ones that do not.
Aerobic quinones further subdivide into two types — ones used by plants to perform photosynthesis and another used by bacteria and animals to breathe oxygen.
“Basically, all forms of life use quinones for their metabolism,” explained Elling. Finding a quinone, “which is similar to what plants use to perform photosynthesis,” in a bacterium that breathes oxygen was highly unusual. Methyl-plastoquinone, the researchers realized, was a third type, and possibly a missing link between the two.
“This molecule is a time capsule. A living fossil of a molecule that has survived over more than 2 billion years.”
Felix Elling
The research sheds light on what is called the Great Oxidation Event. That period — roughly 2.3 to 2.4 billion years ago — marked when cyanobacteria (a type of algae) began generating significant quantities of oxygen as a result of photosynthesis, making aerobic metabolism possible.
While that development would seem to imply that photosynthesis came first, the discovery of methyl-plastoquinone supports another hypothesis. Simply put, some bacteria already had the ability to utilize oxygen — even before cyanobacteria began producing it.
In other words, “the chicken and the egg were at the same time,” Elling said.
Pearson, the PVK Professor of Arts and Sciences and Murray and Martha Ross Professor of Environmental Sciences, in whose lab Elling’s research began, stressed that having a biochemical processing system for oxygen at the advent of its generation by photosynthesis was a huge step.
“The reactions that involve oxygen are very damaging and can be quite deadly to cells that lack mechanisms to cope with the metabolic byproducts,” she said. Although we take them for granted, “the chemical systems that we all employ in our cells to survive our aerobic metabolic lifestyle are actually quite sophisticated.”
Put simply, “this is how we learned to breathe,” Pearson said. “And once you can breathe oxygen and do it safely, it paves the way for the diversification of all the life we see around us.”
Traces of the diversification of quinone structures can be found in our own bodies, including the fundamental distinctions between quinones in human mitochondria, compared to those in plants.
“We think what we found is the primary or ancestral form of this molecule that then later was adapted to have two forms — one with specific functions in the algae and plants, and the alternative form in mitochondria that we have today,” said Elling.
“This molecule is a time capsule,” said Elling. “A living fossil of a molecule that has survived over more than 2 billion years.”
This research was funded in part by the U.S. National Science Foundation.