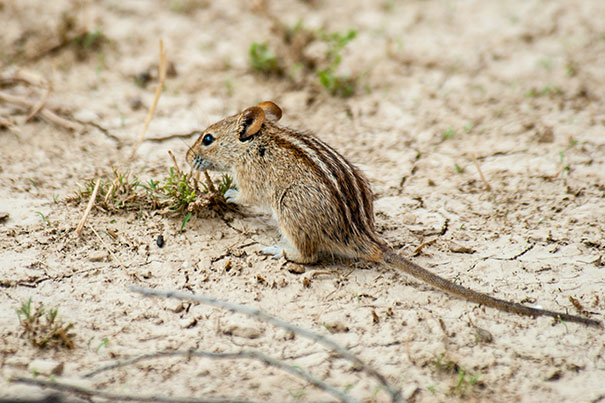
The African striped mouse (pictured) and the eastern chipmunk each have distinctive stripes down their backs that Harvard scientists believe are a byproduct of the cranio-facial gene Alx3 that both species evolved independent of one another.
Photo by Hopi Hoekstra
Science of stripes
Study identifies genetic pathway in African mice, chipmunks
At first glance, it’s easy to think African striped mice and chipmunks might be close cousins. Both are members of the rodent family, both sport distinctive black-and-white “racing stripes,” and both are active during the day.
So how can it be that they actually exist on opposite ends of the rodent family and are separated by some 70 million years of evolution?
The answer, according to a team of Harvard scientists, is that both species use a gene that until now had been associated primarily with cranio-facial development to interrupt the development of pigment cells that form their stripes. The findings are outlined in a Nov. 2 study published in Nature.
More like this
“This is a case where a similar developmental mechanism — the role of this gene — seems to have evolved independently in these two species,” said senior author Hopi Hoekstra, a professor of organismic and evolutionary biology and of molecular and cellular biology, and a Howard Hughes Medical Institute Investigator. “So instead of figuring out a new way to make unpigmented areas, we think evolution may have recycled a protein that already does that job in the belly and expressed that in a new place.
“That gene had previously not been known to affect pigment,” she continued. “We also discovered a new mechanism by which pigment patterns can form, by tinkering with the maturation of melanocytes, or pigment-producing cells. Typically, those cells mature and you can then tinker with what type of pigments are made, but this mechanism is upstream from that.”
Understanding that mechanism would not have been possible were it not for the tools developed in model organisms such as lab mice, said Ricardo Mallarino, a postdoctoral researcher in Hoekstra’s lab and first author of the study.
“What I think is really interesting about our approach is we have one foot in the model species world, and the other in wild rodents displaying natural variation,” he said. “Model species have been very useful, but they can be very isolated from the variation we see in the wild. If we want to learn more about the world, we need to complement model organism research with these non-traditional species … and then we may find new things.”
For Hoekstra and colleagues, the discoveries grew out of a simple question: How did the stripes of the African mouse evolve, and how do they actually form?
When he began looking into those questions, Mallarino found that, though the stripes appear even before the mice are born, the mechanism behind them is unusual.
To produce pigment, Hoekstra explained, animals rely on melanocytes, which migrate around the body during embryogenesis, and are one reason many cats and dogs often sport a white “bib” on their chests — the melanocytes never reached those areas, leaving the hair pigment-free.
To Mallarino’s surprise, though, the white stripes seen on African striped mice weren’t from a lack of melanocytes.
“They are there, so it’s not a migration problem,” Mallarino said. “They get there, but they don’t look fully differentiated, and if they’re not mature they can’t produce pigment, so something is stopping them from maturing.”
To understand what genes might be interfering with the maturation process, Mallarino and colleagues looked at the gene expression patterns in both the black and white stripes, and found a surprising suspect.
“There are all sorts of genes that are more highly expressed in the dark and the light stripes, many of which are classic pigment genes,” Hoekstra said. “But there was one clear outlier in all our analyses, called Alx3. That was a surprise, because I’ve been studying pigment for 15 years, and I’ve never heard of this gene in the context of pigment.”
Earlier studies had shown the gene was linked with cranio-facial deformities such as nasal clefting, but its connection with pigment had gone unnoticed because those studies had been conducted almost entirely in albino mouse strains.
“We decided to follow up on that gene and what we found is it is indeed expressed at high levels in the stripes even before they form, so we had this very beautiful correlative evidence,” Hoekstra said.
Hoekstra and colleagues then conducted a handful of key experiments to over- and under-express the Alx3 gene, and found that its expression was inversely correlated with pigment. When it was highly expressed, pigment was reduced; when its expression was low, pigment increased.
Armed with all the evidence from African striped mice, Hoekstra and colleagues returned to chipmunks to identify whether the same genetic pathway might be involved in their stripes.
“And indeed, Alx3 is also expressed in the white stripes and belly of chipmunks,” she said.
But while both species use a virtually identical genetic pathway to produce their stripes, it’s unlikely the strategy evolved in a common ancestor, Hoekstra said — there is too much diversity separating the two species.
What’s far more likely, she said, is a process biologists call “co-option.” Because Alx3 is involved in creating the white bellies seen in many rodents, evolution simply used a tool that was already at its disposal and expressed the same gene in a new location to create stripes.
In the end, the findings are about more than how two species got their stripes, Mallarino noted.
“The bigger picture here is about understanding how the world works,” he said. “We want to understand how diversity evolves. In the past biologists have wondered about these questions … and they focused on the why. Why does this species have stripes? Does it protect them from predators? To us, an equally interesting question is how. And now we have the tools to dive into the mechanism and understand how this happens, so we can draw some fundamental principles about evolution and how we got here.”
The work was an international collaboration that included researchers from HudsonAlpha Institute for Biotechnology, Stanford University, Instituto de Investigaciones Biomédicas Alberto Sols (CSIC/UAM), CIBER de Diabetes y Enfermedades Metabólicas Asociadas (Ciberdem), Collège de France, University of Strasbourg, and University of the Witwatersrand.