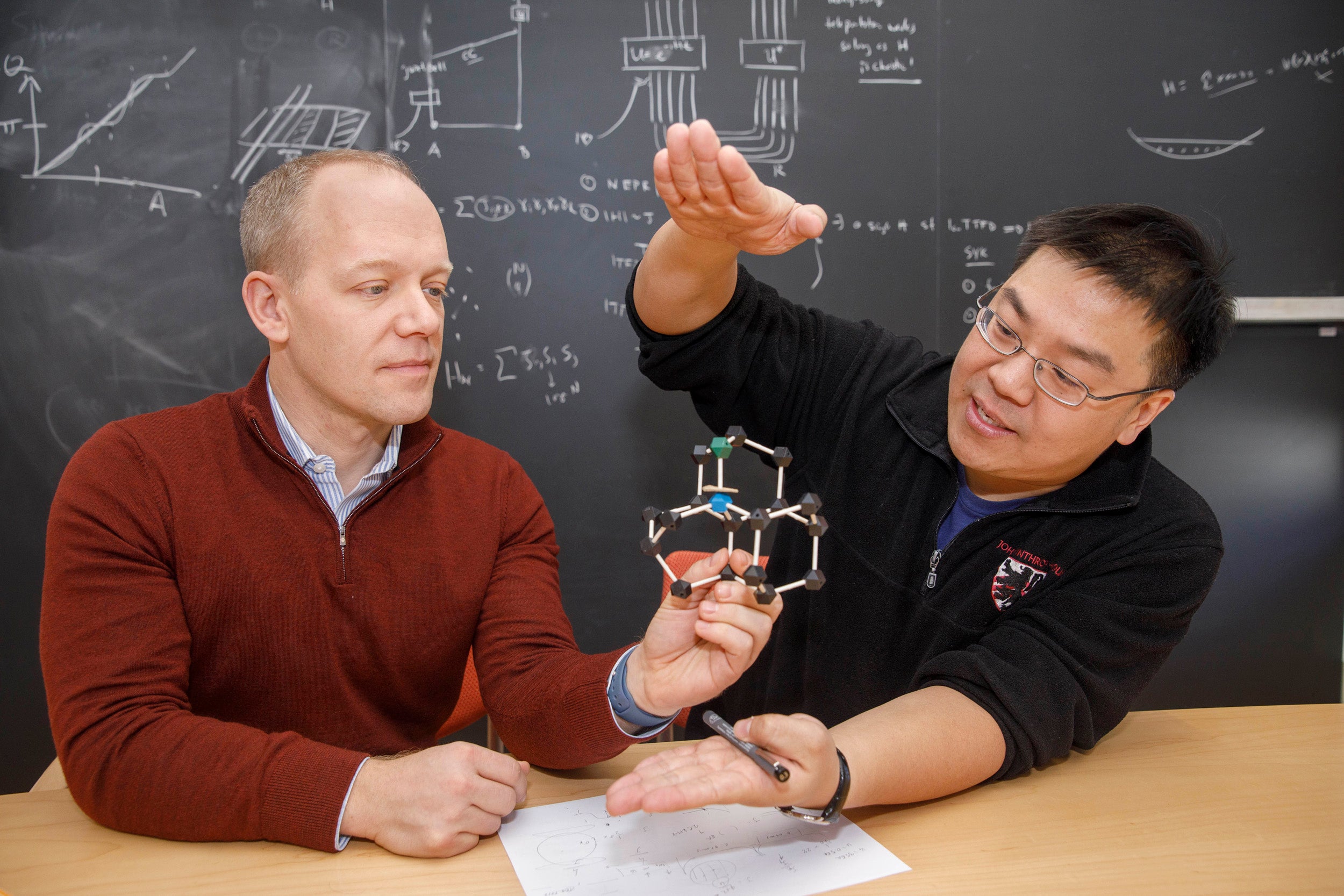
Chris Laumann (left) and Norman Yao explain high-pressure hydride superconductor research.
Kris Snibbe/Harvard Staff Photographer
Hydrogen (like many of us) acts weird under pressure. Theory predicts that when crushed by the weight of more than a million times the Earth’s atmosphere, this light, abundant, normally gaseous element first becomes a metal, and even more strangely, a superconductor — a material that conducts electricity with no resistance.
Scientists have been eager to understand and eventually harness superconducting hydrogen-rich compounds, called hydrides, for practical applications — from levitating trains to electric grids that transmit power with perfect efficiency to new types of electronics and memory devices. But studying the behavior of these and other materials under enormous, sustained pressure is anything but practical, and accurately measuring those behaviors ranges somewhere between a nightmare and impossible.
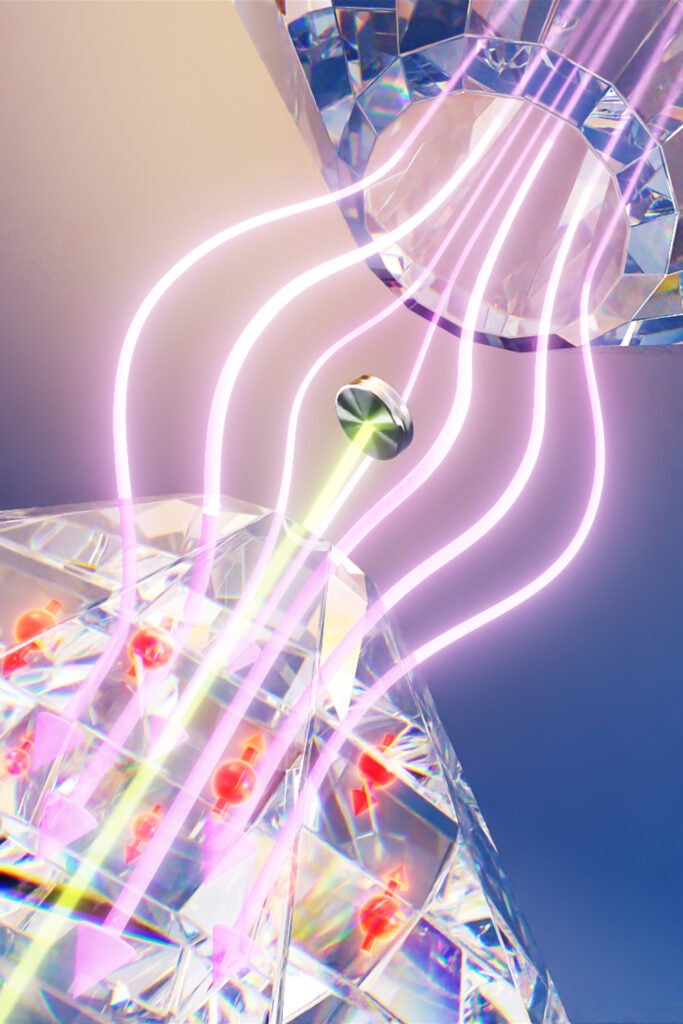
An artist’s rendering of nitrogen vacancy centers in a diamond anvil cell, which can detect the expulsion of magnetic fields by a high-pressure superconductor.
Credit: Ella Marushchenko
Harvard researchers now believe they have a foundational tool for the thorny problem of how to measure and image the behavior of hydride superconductors at high pressure. Published in Nature, they report creatively integrating quantum sensors into a standard pressure-inducing device, enabling direct readouts of the pressurized material’s electrical and magnetic properties.
The innovation came from a longstanding collaboration between Professor of Physics Norman Yao ’09, Ph.D. ’14, and Boston University professor and former Harvard postdoctoral fellow Christopher Laumann ’03, who together broke from their theorist backgrounds into the practical considerations of high-pressure measurement several years ago.
The standard way to study hydrides under extreme pressures is with an instrument called a diamond anvil cell, which squeezes a small amount of material between two brilliant-cut diamond interfaces. To detect when a sample has been squashed enough to go superconducting, physicists typically look for a dual signature: a drop in electrical resistance to zero, as well as the repulsion of any nearby magnetic field, a.k.a. the Meissner Effect. (This is why a ceramic superconductor, when cooled with liquid nitrogen, will hover over a magnet.)
The problem lies in capturing those details. In order to apply the requisite pressure, the sample must be held in place by a gasket that evenly distributes the squishing, then enclosed in a chamber. This makes it hard to “see” what’s happening inside, so physicists have had to use workarounds that involve multiple samples to separately measure different effects.
“The field of superconducting hydrides has been a little bit controversial, partly because the measurement techniques at high pressures are just so limited,” Yao said. “The problem is that you can’t just stick a sensor or a probe inside, because everything’s closed off and at very high pressures. That makes accessing local pieces of information from inside the chamber extremely difficult. As a result, nobody has really observed the dual signatures of superconductivity in a single sample.”
To solve the issue, the researchers designed and tested a clever retrofit: They integrated a thin layer of sensors, made out of naturally occurring defects in the diamond’s atomic crystal lattice, directly onto the surface of the diamond anvil. They then used these effective quantum sensors, called nitrogen vacancy centers, to image regions inside the chamber as the sample is pressurized and crosses into superconducting territory. To prove their concept, they worked with cerium hydride, a material known to become a superconductor at about a million atmospheres of pressure, or what physicists call the megabar regime.
The new tool could help the field not only by enabling discovery of new superconducting hydrides, but also by allowing easier access to those coveted characteristics in existing materials, for continued study.
“You can imagine that because you’re now making something in a [nitrogen vacancy] diamond anvil cell, and you can immediately see that ‘this area is now superconducting, this area is not,’ you could optimize your synthesis and come up with a way to make much better samples,” Laumann said.
The U.S. Department of Energy supported this research.