An assortment of gelatinous biofluorescent and bioluminescent organisms, known as ctenophores and jellyfish, shot on a low-light camera. Biofluorescent organisms absorb light, transform it, and re-emit it as a different color, while bioluminescent organisms create their own light through chemical reactions. Both organism types use their abilities to attract prey or, in some cases, defend themselves from predators. Image by David Gruber, Radcliffe Fellow
Images captured by Harvard researchers often blur the boundary between art and science. From high-powered microscopes to technology that can render biological tissue transparent, new tools are revealing the world in unexpected and compelling ways, expanding our understanding while showcasing unique beauty.
You can see the world in a biofilm, or the universe in a neural network. Jellyfish, seahorses, and turtles fluoresce in the ocean depths, while deadly diseases shimmer virulently under a microscope.
When viewed under the right conditions, the ordinary becomes extraordinary.
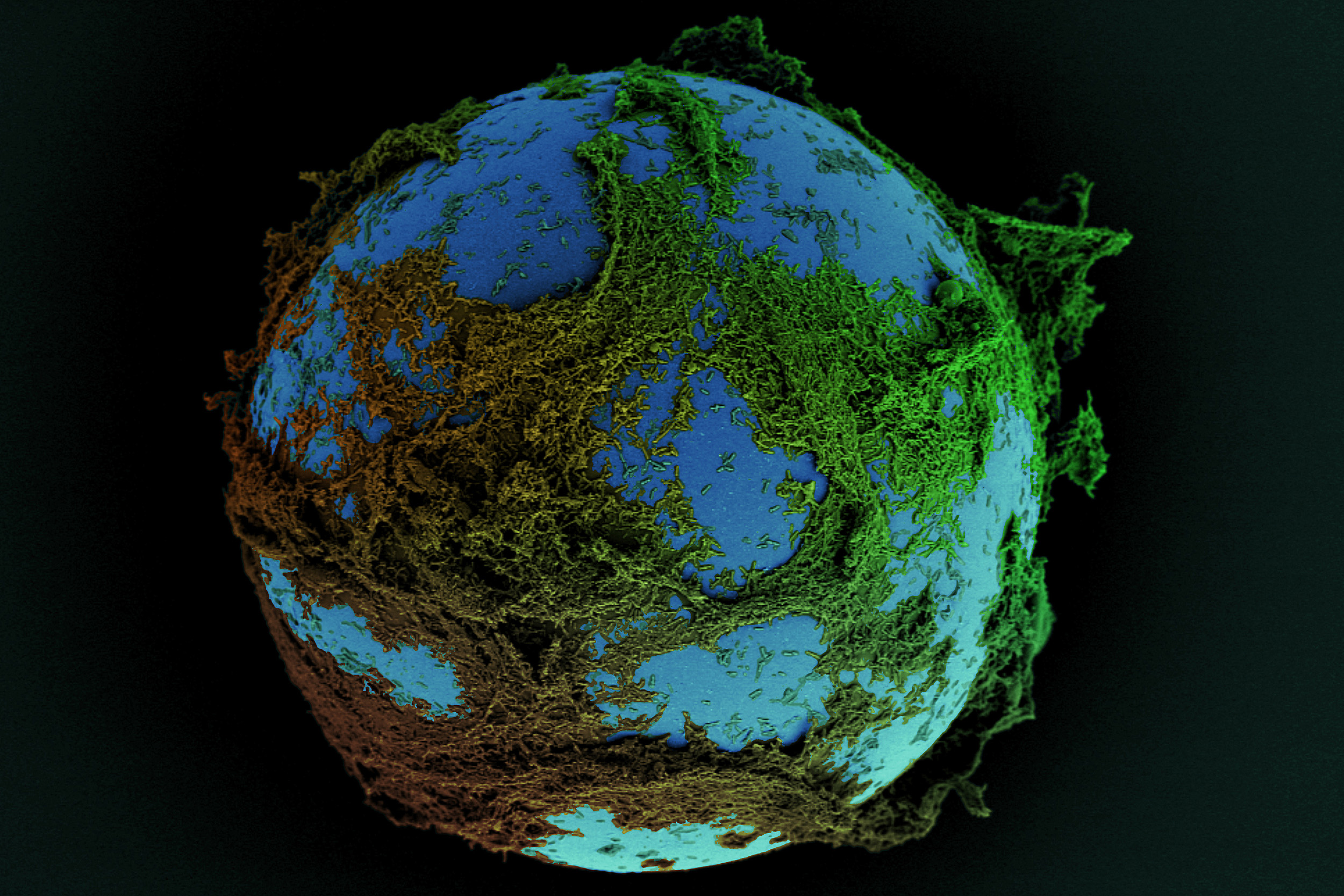
Biomanufacturing is a rapidly growing sector that harnesses biological systems to produce chemicals and materials. In the image above, Wyss Institute researchers have produced decellularized biofilms that can perform complex, biocatalytical manufacturing processes using multiple, individually replenishable enzymes.
Image by Wyss Institute at Harvard University
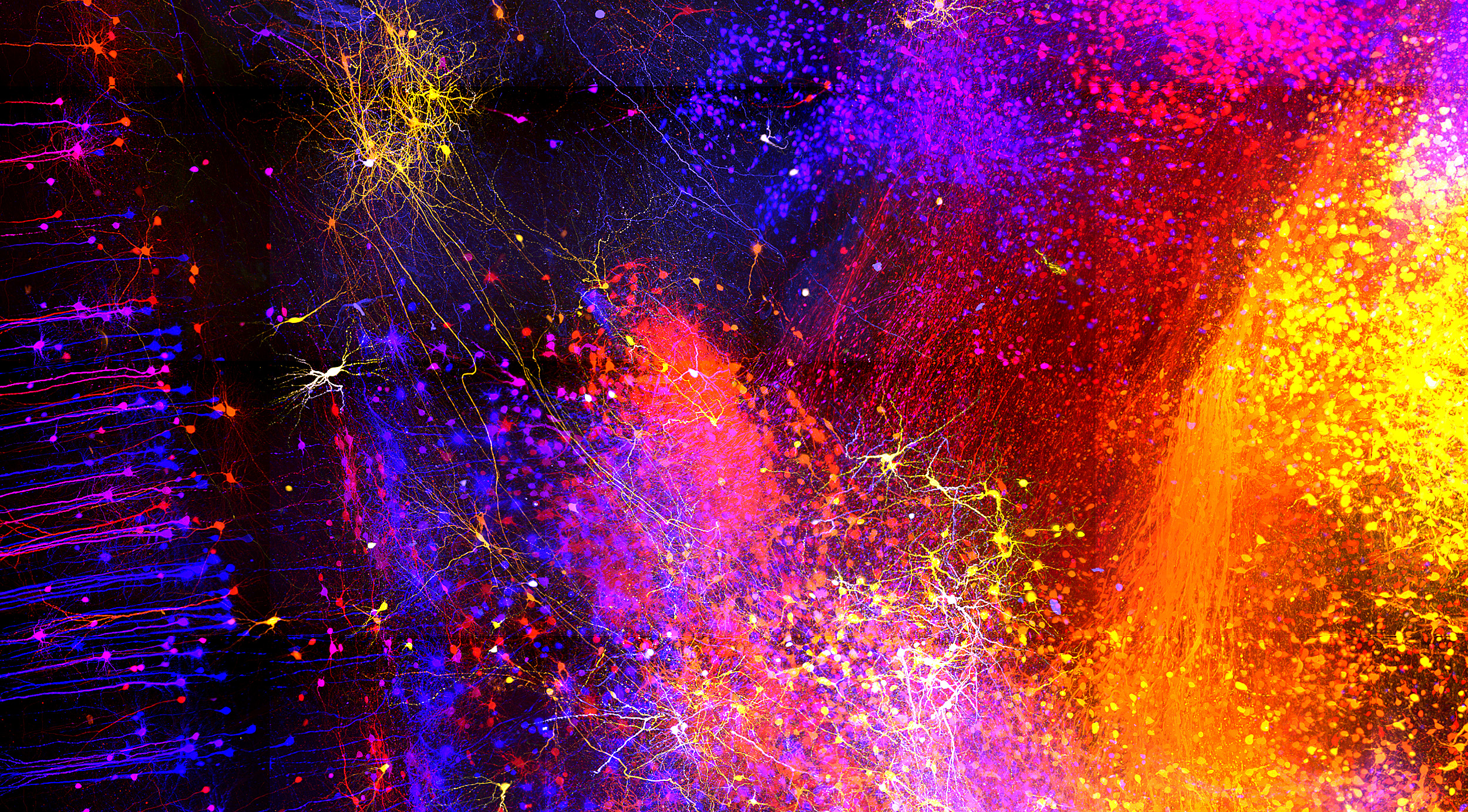
A depth-coded image of all inputs in a large portion of the brain. New molecular tools have revolutionized neuroscience, allowing researchers to label all of the monosynaptic inputs to spatially — and/or genetically — defined populations of neurons, make tissue transparent, and quickly map entire neural circuits.
Image by Will Menegas, Uchida Lab, Department of Molecular and Cellular Biology
Footage of a biofluorescent “glowing” hawksbill sea turtle, captured on camera for the first time. The turtle absorbs light, transforming and re-emitting it as a different color back to the ocean. Scientists are still studying why the turtles, which are critically endangered, emit these lights.
Image by David Gruber, Radcliffe Fellow

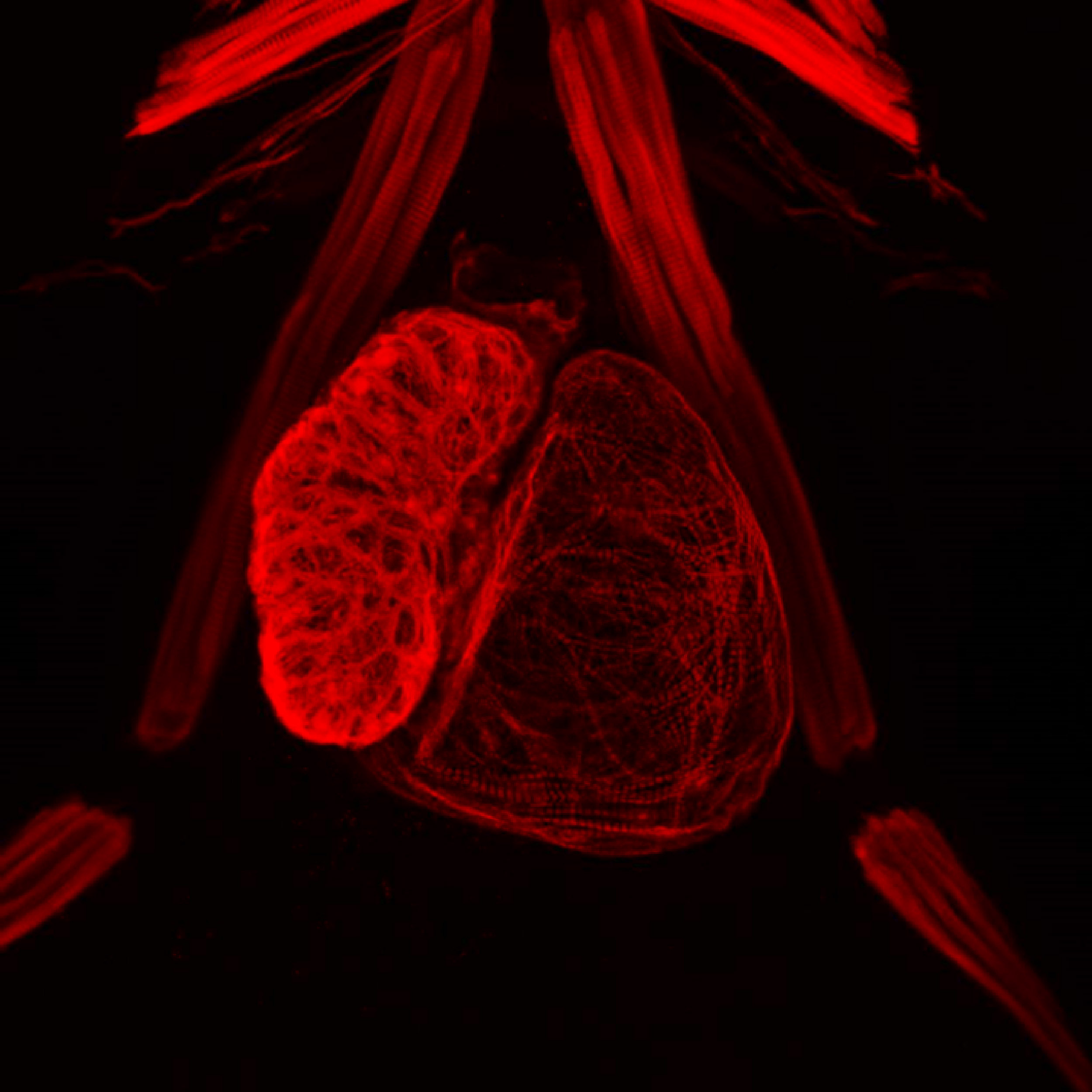
There are a multitude of signals that orchestrate the proper development of the heart. In the first image, the signal labeled in green is important for the formation of cardiac valves in the adult zebrafish, which allow blood to pass from the ventricle (right, orange) to the atrium (left, orange). Unlike mammalian hearts, which have four chambers, the zebrafish heart consists of only two: a single ventricle (left) and a single atrium (right). The second image was created to help researchers visualize the muscles in the heart that allow it to pump.
Images by Michka Sharpe, Burns Lab, Biological and Biomedical Sciences (BBS) Program, Harvard Medical School
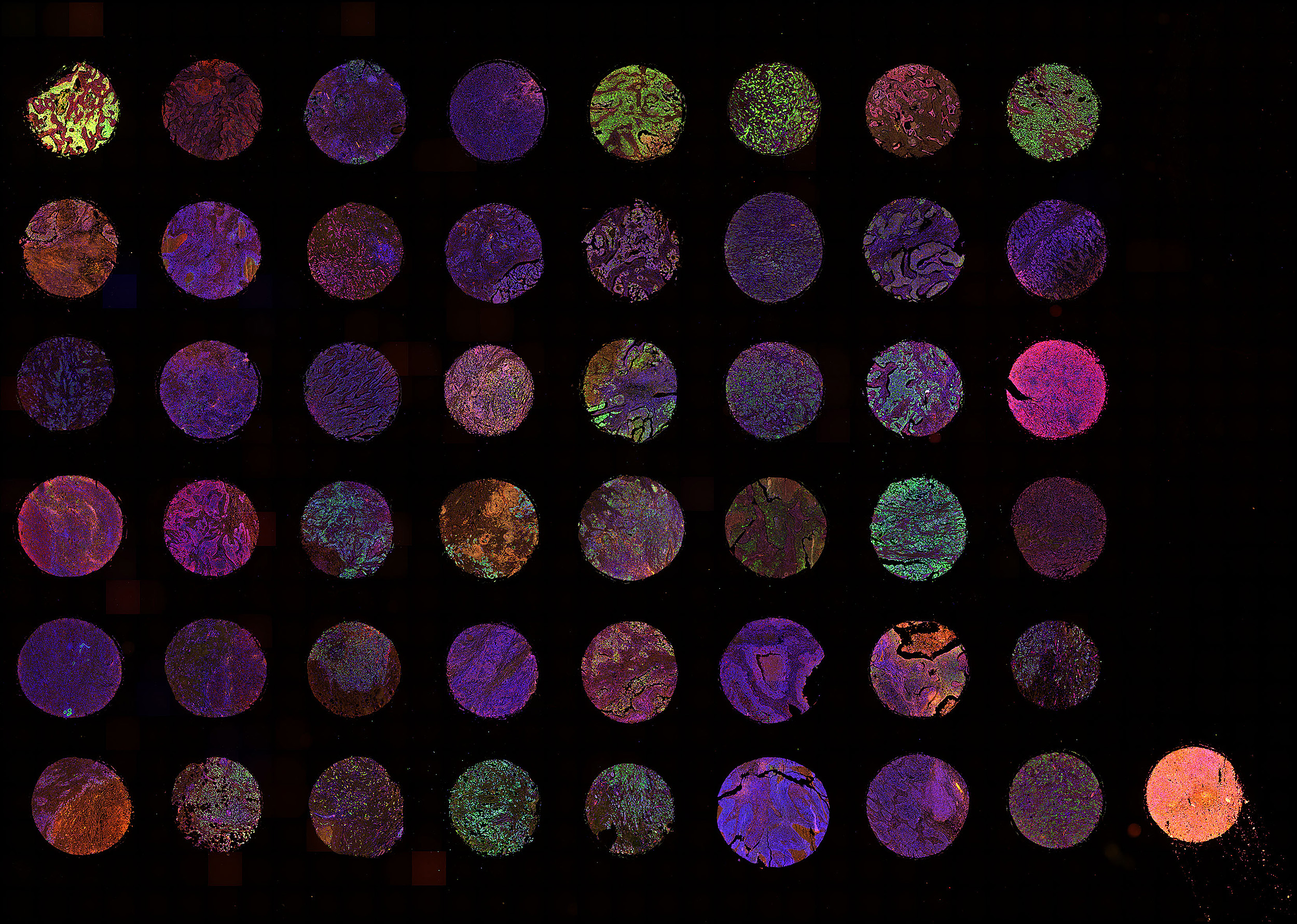
Biopsies from more than 40 tumors were arranged in vertical “posts” in a wax block, sliced into thin sections, and placed on microscope slides. Antibodies were attached to four molecules of interest, allowing researchers to rapidly scan multiple biomarkers over hundreds of tumors and establishing molecular “signatures” while monitoring variability within a single tumor type.
Image by Douglas Richardson, Harvard Center for Biological Imaging, Department of Molecular and Cellular Biology

Closeup of a triplefin blenny taken in the Solomon Islands using a stereo fluorescent microscope. This image was part of a study that identified more than 180 new species of biofluorescent fish and helped initiate biofluorescence as a significant area of marine biology. These fish are hard to see on a reef under normal light conditions, but glow vibrantly when illuminated with the fluorescent microscope.
Image by David Gruber, Radcliffe Fellow
Excitatory neurons (red) and the inhibitory neurons (green) of a transgenic zebrafish larva. Labeling subpopulations of neurons in color allows scientists to monitor their activity and understand their role in neural circuits.
Image by Abhinav Grama, Cox Laboratory, Department of Molecular and Cellular Biology

Muscle fiber. YAP protein (red) is known to be a key mediator of cellular growth, differentiation, and survival in many systems, such as mammalian muscle, and acts as a sensor, integrating mechanical cues with gene expression.
Image by Margarete Diaz Cuadros, Whited Lab, Biological and Biomedical Sciences (BBS) Program, Harvard Medical School

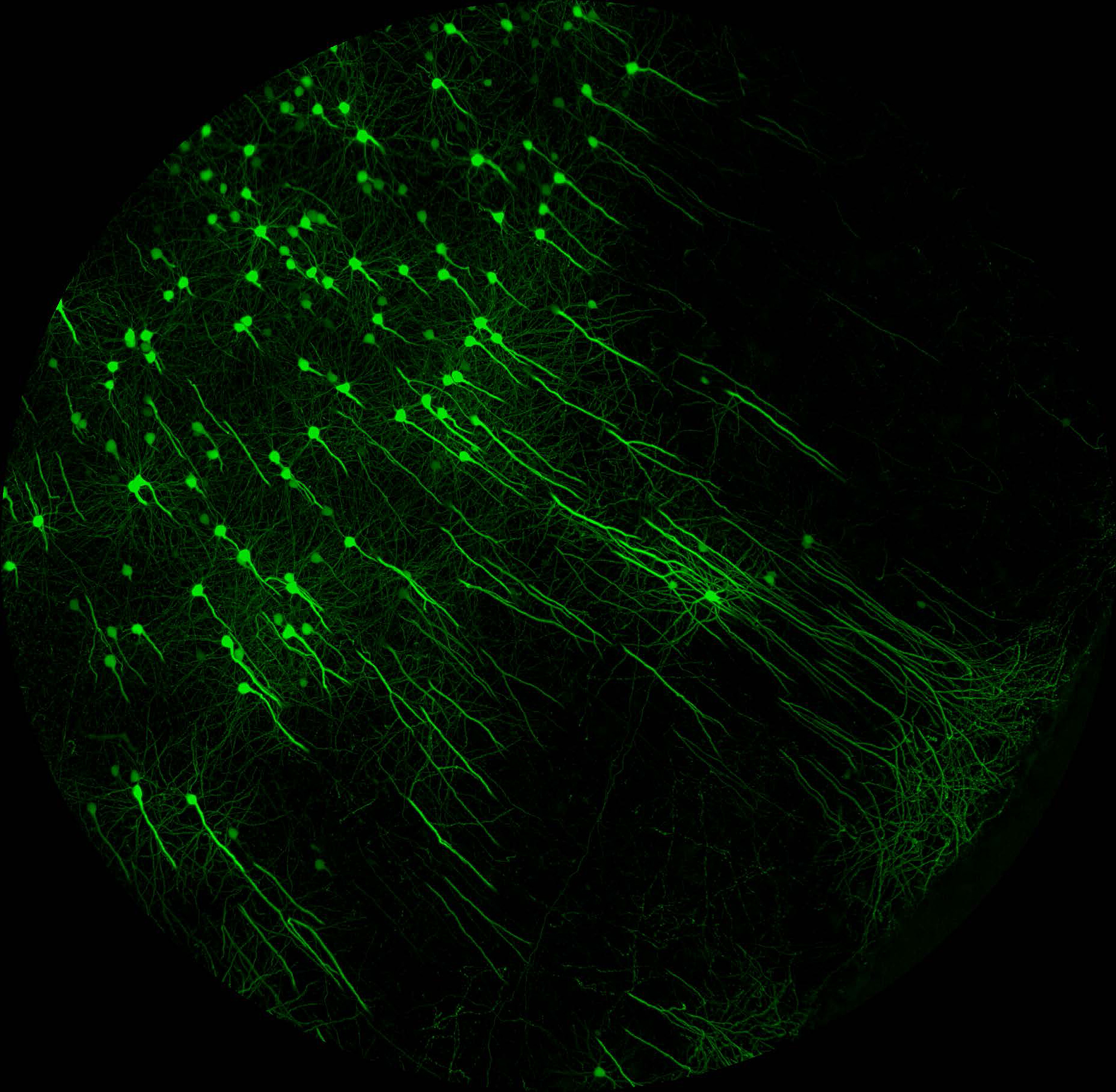
Photo 1: A calcium indicator (green) allows scientists to record the activity of neurons in the primary visual cortex and determine the state of the brain’s neural network in response to different visual stimuli. Photo 2: A number of new chemical treatments have been developed by researchers that preserve the protein structure of biological tissue while turning it transparent, allowing specialized microscopes to image neurons through many millimeters of intact tissue.
Image 1 by Abhinav Grama and David Mankus, Cox Laboratory, Department of Molecular and Cellular Biology; Image 2 Douglas Richardson, Harvard Center for Biological Imaging, Department of Molecular and Cellular Biology
C. elegans, a nematode worm commonly found in soil and compost across the globe, only lives for a few weeks but during that short time displays obvious signs of aging, making it an ideal tool for studying the molecular biology of aging.
Image by Mair Lab, Harvard School of Public Health

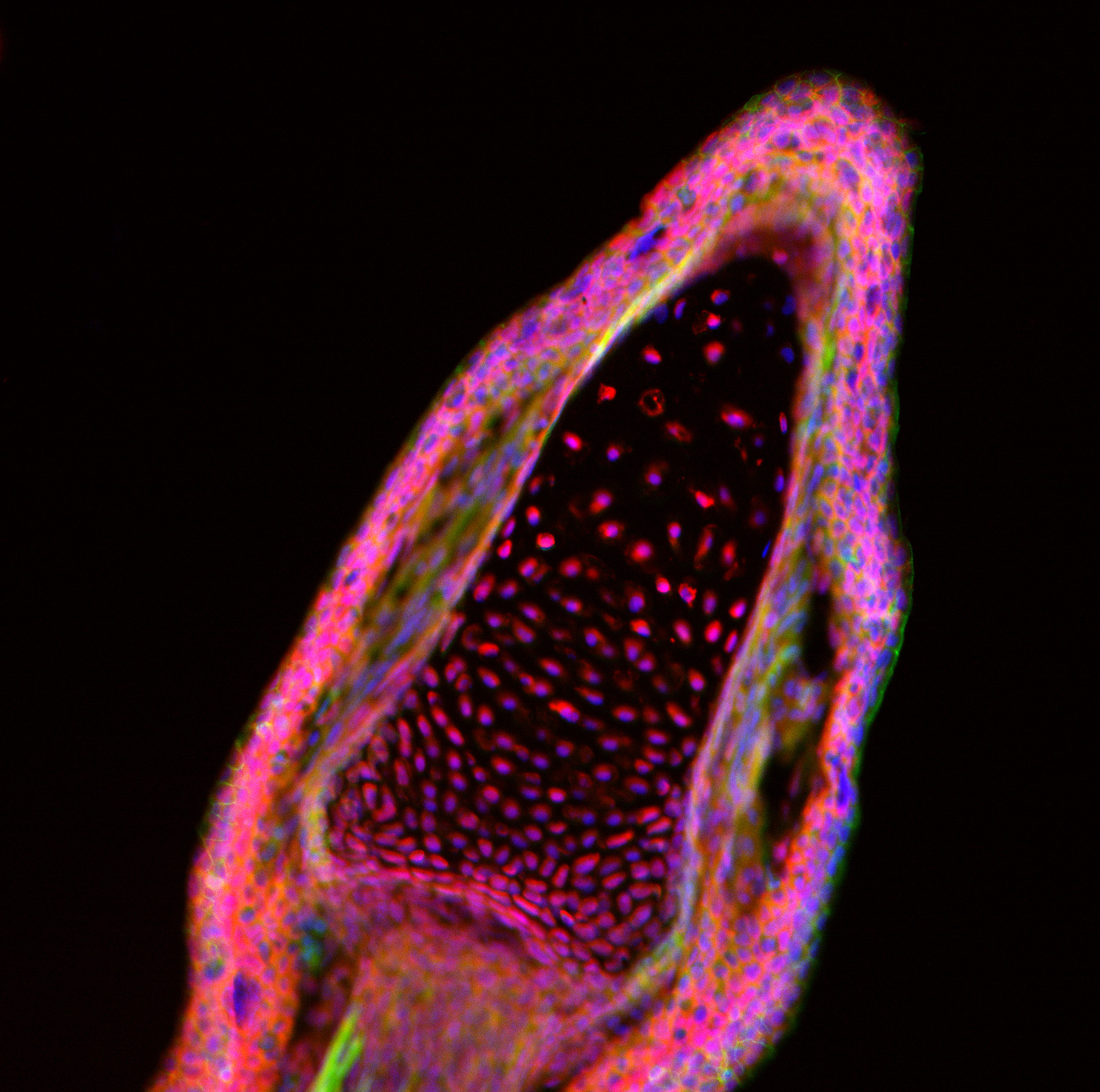
Fingertip of the axolotl salamander. Cell nuclei (blue), a component of the cell’s cytoskeleton known as filamentous actin (green) and YAP protein (red), a component of a key signaling pathway in development and growth.
Image by Margarete Diaz Cuadros, Whited Lab, Biological and Biomedical Sciences (BBS) Program, Harvard Medical School
A developing zebrafish eye three days after fertilization. The dark circle that appears to be a hole in the middle of the eye is the zebrafish lens, the part of the eye that bends light, allowing zebrafish (and other vertebrates) to see distant objects sharply.
Image by Michka Sharpe, Burns Lab, Biological and Biomedical Sciences (BBS) Program, Harvard Medical School

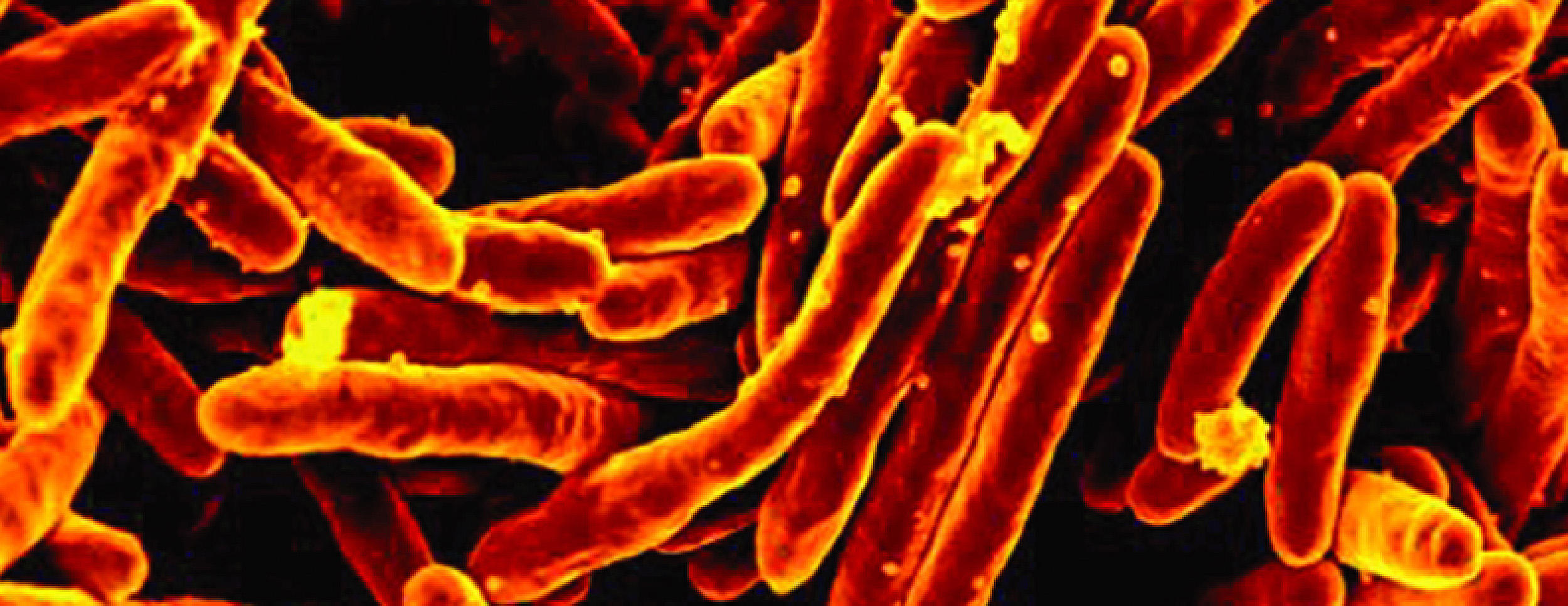
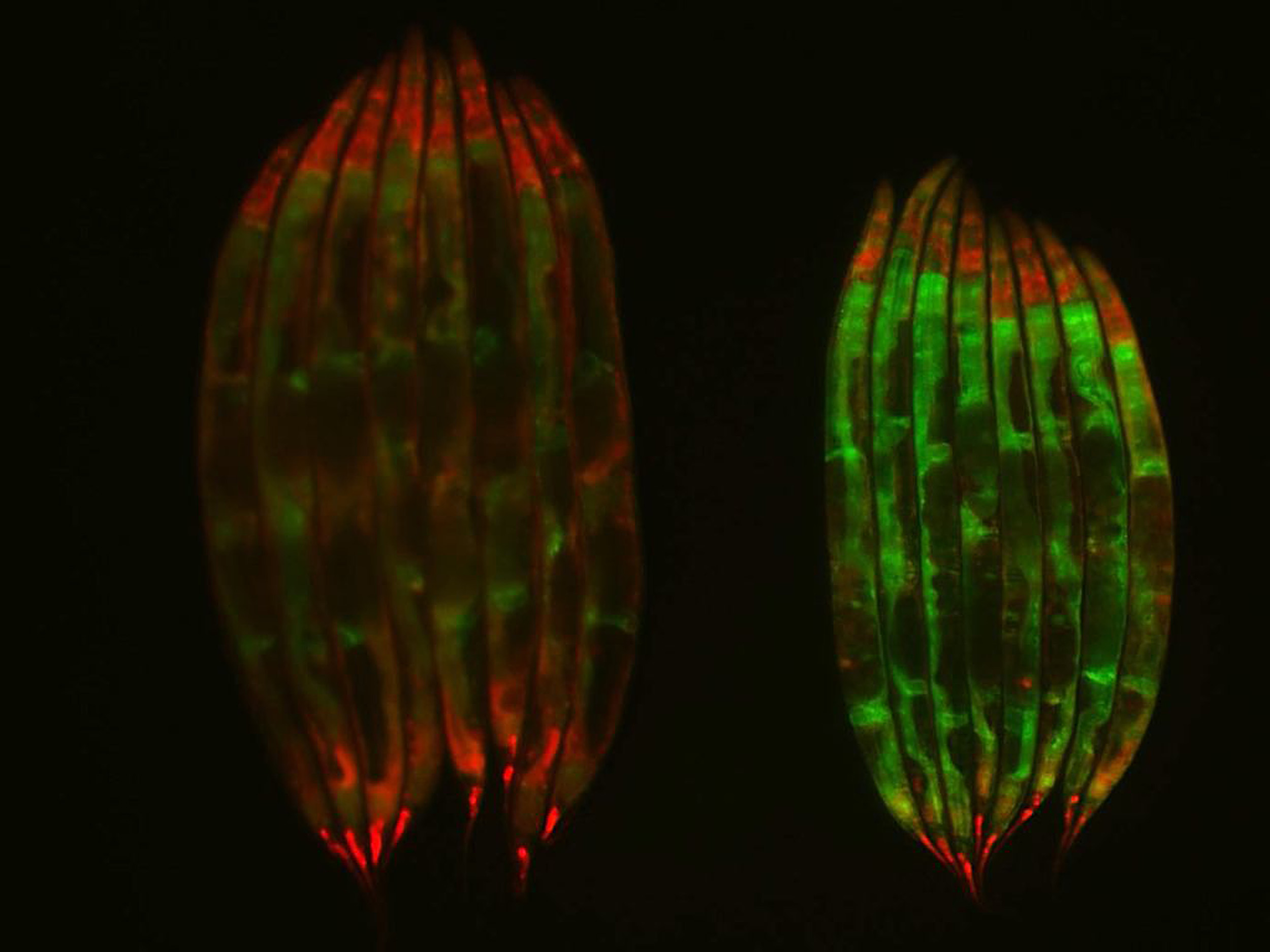
Image 1: Despite onslaughts from the immune system and drug treatments, tuberculosis kills 1.5 million people each year. To understand how cells respond to tuberculosis infection, researchers wield the latest imaging equipment to visualize microbes, employ advanced genomic methods to trace individual bacterial cells, and cut through an ever-increasing amount of genomic data with powerful mathematical tools. Image 2: Researchers found that c. elegans worms on dietary restriction maintained a youthful splicing pattern (as seen in young worms) compared to the well-fed worm population at the same age. In this image, the worms on the left are well-fed, while the ones on the right have undergone dietary restriction.
Image 1 by Sarah Fortune, John LaPorte Given Professor of Immunology and Infectious Diseases, Harvard School of Public Health; Image 2 Caroline Heintz, Mair Lab, Harvard School of Public Health
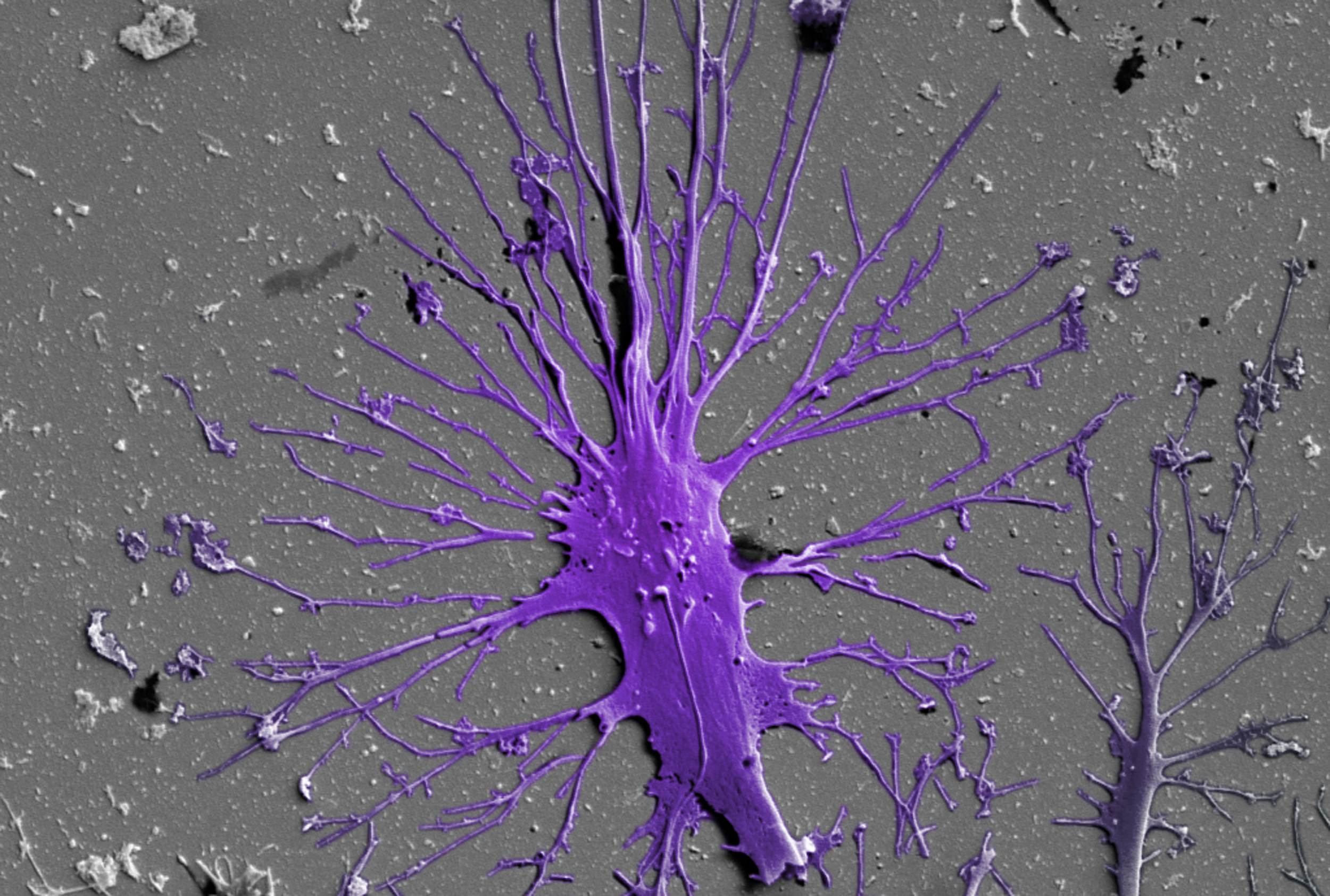
Podocytes, an essential part of the kidney’s filtration system, are often targeted by kidney diseases and harmed by drugs. To better understand how podocytes function, researchers at the Wyss Institute created a biological model of the cell. Image: Scanning electron micrograph of a human stem cell-derived matured podocyte with multiple primary and secondary processes extending in all directions on extracellular matrix-coated material.
Image by Wyss Institute at Harvard University