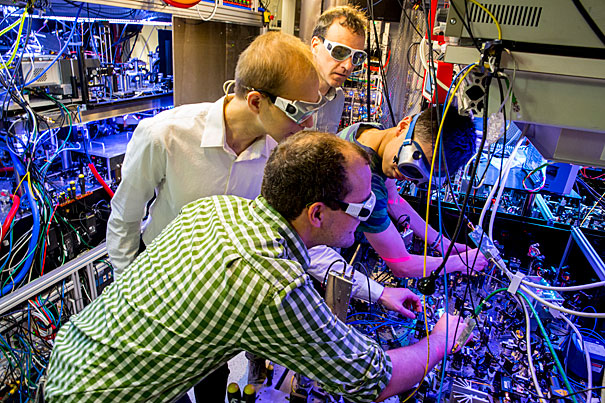
Physics Professor Markus Greiner (in back) and his team — Anton Mazurenko (from left), senior grad student, Daniel Greif, postdoc fellow, Geoffrey Ji, graduate student, and Christie Chiu (not shown) — have created an antiferromagnet, an exotic form of matter that can give important insights into how room temperature superconductors might be created.
Rose Lincoln/Harvard Staff Photographer
Figuring out superconductors
Physicists create antiferromagnet that may help develop, monitor key materials
From the moment when physicists discovered superconductors — materials that conduct electricity without resistance at extremely low temperatures — they wondered whether they might be able to develop materials that exhibit the same properties at warmer temperatures.
The key to doing so, a group of Harvard scientists say, may lie in another exotic material known as an antiferromagnet.
Led by physics professor Markus Greiner, a team of physicists has taken a crucial step toward understanding those materials by creating a quantum antiferromagnet from an ultracold gas of hundreds of lithium atoms. The work is described in a May 25 paper published in the journal Nature.
“We have created a model system for real materials … and now, for the first time, we can study this model system in a regime where classical computers get to their limit,” Greiner said. “Now, we can poke and prod our antiferromagnet. It’s a beautifully tunable system, and we can even freeze time to take a snapshot of where the atoms are. That’s something you won’t be able to do with an actual solid.”
But what, exactly, is an antiferromagnet?
Traditional magnets, the kind that you can stick to your refrigerator, work because the electron spins in the material are aligned, allowing them to work in unison. In an antiferromagnet, however, those spins are arranged in a checkerboard pattern. One spin may be pointed north, while the next is pointing south, and so on.
Understanding antiferromagnets is important, Greiner and physics professor Eugene Demler said, because experimental work has suggested that, in the most promising high-temperature superconductors — a class of copper-containing compounds known as cuprates — the unusual state may be a precursor to high-temperature superconductivity.
Currently, Demler said, the best cuprates display superconductivity at about minus 160 degrees Fahrenheit, which is cold by everyday standards, but far higher than for any other type of superconductor. That temperature is also warm enough to allow practical applications of cuprate superconductors in telecommunications, transportation, and in the generation and transmission of electric power.
“This antiferromagnet stage is a crucial stepping-stone for understanding superconductors,” said Demler, who led the team providing theoretical support for the experiments. “Understanding the physics of these doped antiferromagnets may be the key to high-temperature superconductivity.”
To build one, Greiner and his team trapped a cloud of lithium atoms in a vacuum and then used a technique they dubbed “entropy redistribution” to cool them to just 10 billionths of a degree above absolute zero, which allowed them to observe the unusual physics of antiferromagnets.
“We have full control over every atom in our experiment,” said Daniel Greif, the postdoctoral fellow working in Greiner’s lab. “We use this control to implement a new cooling scheme, which allows us to reach the lowest temperatures so far in such systems.”
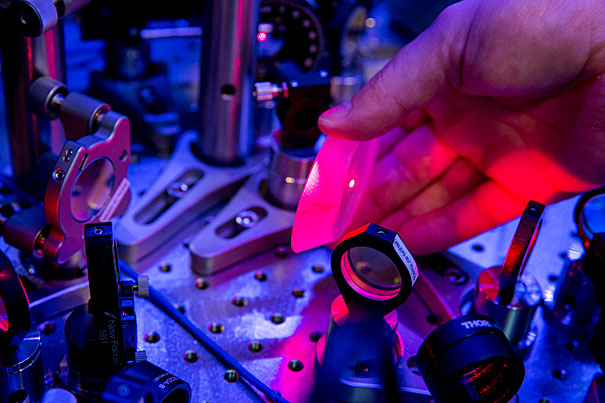
That degree of control has enabled Greiner and his team to photograph the system with enough detail to identify and extract information about individual atoms. The team can also change the atomic density of the antiferromagnet to search for a superconducting state.
The system isn’t just a model, but is a special-purpose quantum computer that can simulate the complex physics of antiferromagnets and how their transformation into superconductors can work.
Though scientists can simulate the quantum properties of simple atoms, and even relatively simple materials, more exotic compounds like cuprates are simply too complex to be modeled accurately by conventional computers, and many in the field believe that quantum computers may be the answer.
“Many people expect that the first field where quantum computers will make a major impact is in quantum simulation,” Demler said. “If scientists want to test the airflow and other flight characteristics of an airplane, they would build a wind tunnel to test that. This is, essentially, a quantum wind tunnel for real materials.
“So what we have done in the past is to come up with what we think are simple models. The truth is we still cannot solve those models,” Demler said. “The end result is that our predictions disagree with experimental results, but we don’t know if our model was incorrect or if we didn’t compute it correctly. With this system, we know exactly which model describes it. And now … if we make a prediction, they can tell us if it is accurate.”
Though the system may one day play a role in designing a new generation of superconductors, Demler said its ultimate importance may lie in helping researchers build a foundation of knowledge for materials science.
“The problem in trying to come up with better superconductors is that if you take a material and change one parameter … lots of things are changing,” Demler said. “With this simulation, we have full control of parameters. So we can actually understand what helps and what suppresses superconductivity, and then we can become wiser in terms of choosing elements” to investigate.
This research was supported by the Air Force Office of Scientific Research, Army Research Office, the Gordon and Betty Moore Foundation EPiQS Initiative, the Harvard Quantum Optics Center, the National Science Foundation and the Swiss National Science Foundation.