Back into the dark
With collider set to reboot, physicists look beyond the Higgs
So much for the warmup laps.
Harvard physicists are looking with anticipation to the spring, when the Large Hadron Collider (LHC) at CERN, Switzerland, fires up after a two-year hiatus for repairs and upgrades. The last time it was running, science celebrated the discovery of the Higgs boson, a long-sought elementary particle and the last one predicted by the Standard Model.
There may be a lot more to come. The Higgs discovery was made before the LHC ever got to full power. It was running at just 8 teraelectron volts (TeV) — higher than any collider had ever run, but far short of its designed 14 TeV maximum.
The upgrades and repairs since early 2013 are expected to allow the collider to run at 13 TeV. The results are anybody’s guess.
“That’s what’s exciting about it,” said Mallinckrodt Professor of Physics Melissa Franklin. “If we knew what we were going to find, we’d all just go home.”
Physicists knew that the LHC, the most powerful collider in history, would take them to a new energy frontier as it began operating in 2008. At the same time, they had a pretty good sense of what they’d find.
The Standard Model of physics had proven remarkably durable over the preceding decades. One by one, experimentalists had found each of the 40 particles it predicted — bottom quark, top quark, W and Z bosons. All save one: the Higgs boson.
The discovery would prove that the theorized Higgs field, present throughout the universe, actually existed. The particle was believed to be the physical manifestation of that field, and to carry the force that gives particles mass. The find would also explain why some particles were heavier than others: They interacted more strongly with the Higgs boson.
So, with decades of theorizing behind them and the prior particle discoveries as evidence that the Standard Model was correct, physicists were pretty sure the Higgs boson was out there.
But now …
“It’s sort of like you’re Arctic explorers, and though you knew that there were some milestones to be seen or predicted, you’ve gone past that. Now you’re really into the unknown at this point and one doesn’t really know what to expect,” said Donner Professor of Science John Huth, who has been involved since 1995 with the Large Hadron Collider’s ATLAS experiment.
Mysteries abound
Though the Higgs boson completes the Standard Model, that doesn’t mean there’s nothing left to find — far from it. “Out there” remains an open question, mostly.
Current models hold that the stuff we know about — ourselves, our cars, our houses, the solar system, interstellar dust, etc. — makes up just about 5 percent of the universe. A big chunk of the rest, 27 percent, is something called dark matter, whose gravitational effects astrophysicists see as they peer into the skies, but whose nature remains a mystery. The remainder — roughly 68 percent — is dark energy, about which scientists understand even less. (Chris Stubbs, the Samuel C. Moncher Professor of Physics and of Astronomy, is one of the number who are on the case, albeit at the Large Synoptic Survey Telescope in Chile rather than the LHC.)
Other mysteries include how gravity is related to the other three main forces in the universe: electromagnetism and the strong and weak forces that operate in the atomic nucleus. Today they’re explained by separate theories. A theorized particle, the graviton, that might carry the gravitational force, has never been seen. There’s also the question of whether supersymmetry — thought to include a whole new family of particles — is real. And then there’s the possibility that the Higgs boson hasn’t been fully described.
“It’s both exciting and scary because, on one hand, we could [find] significant, really new physics that could explain a heck of a lot and all of a sudden the pieces line up in terms of cosmology,” Huth said. “On the other hand, [we could find that] everything works out exactly as predicted, that there’s nothing new and you’re left with, ‘Now what?’ You put a lot into it, and you’d like to see something come out, but as an experimentalist, you have to let Mother Nature tell you what’s out there.”
Huth and his Harvard colleagues are ready to listen. He’d like to hear in particular what else she has to say about the Higgs, as would Franklin and Associate Professor Joao Guimaraes da Costa. Masahiro Morii, chair of the Physics Department, is especially interested in dark matter and supersymmetry.
ATLAS will no doubt be a factor in their investigations. The experiment — a collaboration of 3,000 scientists in 38 countries in which Harvard physicists have played a leading role — has been a hub for the four University faculty members, six postdoctoral fellows, and 11 graduate students connected to the LHC.
ATLAS — “a toroidal LHC apparatus” — is one of four enormous detectors arranged around the LHC’s 17-mile underground ring. When operating, the LHC accelerates beams of protons in opposite directions and crosses them inside ATLAS. The collisions happen so rapidly and generate so much data that scientists have to apply filters and examine only the most promising results. Even so, there are 200 such “events” each second, generating an enormous amount of information for analysis, an amount equal each year to 160 times the volume of books in the Library of Congress, according to ATLAS statistics.
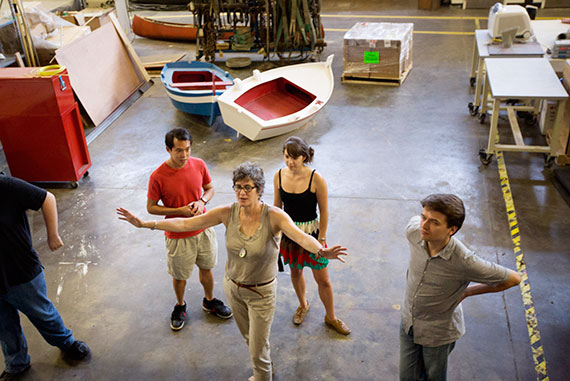
Onward to supersymmetry
Having established the existence of the Higgs, physicists plan to use the LHC’s higher energy to probe the particle’s properties, including how it couples to other particles, whether there are multiple kinds of Higgs bosons, why it is so light, and how often it decays into different particles. The upgraded collider should generate particles more rapidly in the years to come and thus create more opportunities for study.
“There is no doubt that there is a particle we found that has the characteristics of and behaves like the Higgs boson,” Guimaraes said. “But it’s not exactly the Higgs boson described in the Standard Model.”
“We have only measured some properties and we haven’t measured them well,” said postdoctoral fellow David López Mateos, who is working with Huth. “We have a duty to [find out] that it is the Higgs of the Standard Model, and we may find some new physics along the way.”
The Higgs will also be used as a tool to bring other secrets to light, said physicist Chris Rogan, a junior fellow in Harvard’s Society of Fellows. Because certain particles interact with partner particles — think protons and electrons — the revelation of an unknown particle that interacts with the Higgs is possible.
“There are all sorts of unanswered questions. We don’t know what the answers are going to be, but we do know they lie at higher energy scales,” Rogan said.
Supersymmetry, dating to the 1960s, is a theory of the universe that builds on the Standard Model. It suggests that each of the known particles once had a symmetric partner that looked a lot like it, save for the property of spin. Somehow, in a fraction of the universe’s first second, that initial symmetry broke, changing the properties of the partner particles. One of the changes was that they became heavier, meaning they can only be produced in collisions more powerful than any we’ve seen so far.
Some scientists consider supersymmetry a possible successor to the Standard Model because it would fill holes in our understanding of how the universe works. When the collider starts up again in the spring, Morii and Rogan will be among those on the lookout for symmetric partner particles — squarks, gluinos, and others.
“It really could solve a lot of problems all at once,” Rogan said.
A prime candidate, Morii said, would be the supersymmetric partner to the top quark, called the top squark, because the Higgs couples more strongly to heavier particles and the top quark is the heaviest.
Others, though, aren’t so sure about supersymmetry. Guimaraes said it is a bit too neat to be believed.
“It’s a pretty theory, so people want it, but there’s lots of pretty things that don’t exist,” Guimaraes said. “As an experimentalist, you have to go with what you see.”
One area of consensus is that a new understanding of dark matter may be in the offing. Unlike the search for evidence of supersymmetry, the search for dark matter has the advantage of physicists knowing it exists. That’s because astrophysicists have detected the gravitational effects of an enormous amount of invisible matter in the universe tugging on the things that they can see.
So physicists are rummaging in their bag of tricks for ways to detect an almost invisible particle — one that doesn’t interact with any known particle, is heavy enough that it hasn’t been generated before, and is very stable, with almost infinite life.
“It can’t be too hard to make or there wouldn’t have been a lot made in the Big Bang,” Morii said.
One way to search would be through “monojets,” or single particles shooting off at an angle, apparently from a collision with an undetected particle. The laws of physics dictate that the particle must have bounced off something — a clue that investigators such as postdoctoral fellow Valerio Ippolito are studying more closely.
It’s even possible, according to Morii, that researchers will kill two birds with one stone, tracing dark matter to a supersymmetric particle, something called a neutralino.
“In a sense, we are at a point where there is no one thing that we know has to exist,” Morii said. “Dark matter has to exist, but there’s many different possible shapes. Higgs mass stabilization has to happen, but supersymmetry is only one possible explanation. … We are searching, but we know very well that we’re searching for something that doesn’t have to exist.”
Indeed, there are no guarantees in physics. Franklin cautioned that a higher-energy beam doesn’t necessarily mean that new discoveries are around the corner. There have been large droughts between particle breakthroughs despite increasingly powerful accelerators, she pointed out. The discovery in 1995 of the top quark at Fermilab — an effort both Huth and Franklin were involved in — was preceded by 12 particle-less years, after the W and Z bosons were discovered in 1983.
Learning while doing
Though activity at the LHC is focused on research, the work also helps train graduate and undergraduate students. Huth and Franklin said the educational opportunities include hands-on construction as well as the kind done on computers.
ATLAS and the LHC have been as much a part of doctoral student Tomo Lazovich’s Harvard experience as House life and the Yard. He began working with Franklin at Fermilab in Illinois the summer after his freshman year in 2008. The following summer, he went to CERN for the first time to work on commissioning the ATLAS detector.
Lazovich graduated from the College in 2011 and started graduate school that fall. He worked on the rapid decay of Higgs particles into two W bosons, an important process to understand because the Higgs, when it can be produced, exists for only a fraction of a second before it decays into other particles. It is through detailed analysis of these “decay paths” that scientists detect the Higgs.
Lazovich plans to be on hand when the collider restarts.
“It’s been amazing just having the intellectual experience. Even the possibility of coming to Harvard as a high school student seemed a remote thing. The whole experience has been surreal.”
Nothing will be revealed without a lot of hard work. Faculty members, fellows, and students are immersed in prep work.
The upgrades, as big as everything else to do with LHC, are being carried out by investigators and students from 15 institutions, including Harvard, Brookhaven National Laboratory, the University of Arizona, and the Weitzmann Institute of Science.
Harvard’s portion of the job is work on the muon detector at ATLAS’ heart, a hulking cube in a bay at 38 Oxford St. Electronics are being refined at offices in nearby Palfrey House.
“That’s so great in terms of people learning,” Franklin said. “It’s nice having the undergraduates do real stuff, and not just work on the computer.”
Meanwhile, the spring’s “first beam” draws nearer.
“I see the last run almost as a test run,” said Rogan. “The real opportunities for discovery are right around the corner. If any new physics live just beyond our reach, it could pop out quite quickly, which would be very exciting and which is why we’re expending a lot of effort making sure we’re ready.”