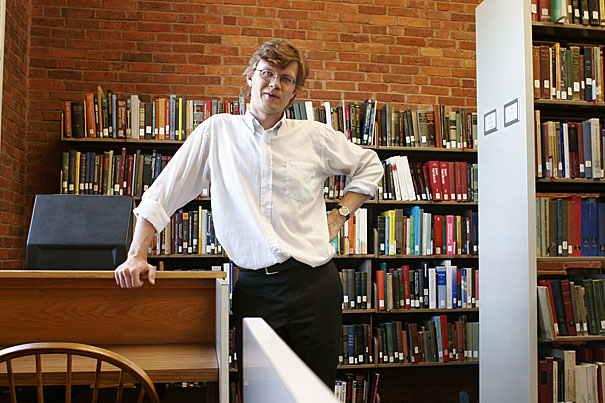
A team of Harvard physicists, led by Mikhail D. Lukin (pictured), have achieved the first quantum entanglement of photons and solid-state materials.
File Rose Lincoln/Harvard Staff Photographer
Quantum connections
Harvard physicists show how bits interact over long distances
Harvard physicists have achieved the first quantum entanglement of photons and solid-state materials. Their achievement marks a key advance toward practical quantum networks, as the first experimental demonstration of a means by which quantum bits, or “qubits,” can communicate with one another over great distances.
A team led by physicist Mikhail D. Lukin reported the findings in the latest edition of the journal Nature.
“In quantum computing and quantum communication, a big question has been whether or how it would be possible to actually connect qubits, separated by long distances, to one another,” said Lukin, a professor of physics at Harvard. “As the first demonstration of quantum entanglement between a solid-state material and photons, our work is an important advance toward linking qubits together into a quantum network.”
Quantum networking applications such as long-distance communication and distributed computing (in which computers interact through a network) would require the nodes that process and store quantum data in qubits to be connected to one another by entanglement: a state where two atoms become indelibly linked so that one inherits the properties of the other. This state has previously been demonstrated only with photons and individual ions or atoms.
“Our work takes this one step further, showing how one can engineer and control the interaction between individual photons and matter in a solid-state material,” said first author Emre Togan, a Harvard graduate student in physics. “What’s more, we show that the photons can be imprinted with the information stored in a qubit.”
Quantum entanglement, famously termed “spooky action at a distance” by a skeptical Albert Einstein, is a fundamental property of quantum mechanics. It allows distribution of quantum information over tens of thousands of kilometers, limited only by how fast and how far members of the entangled pair can propagate in space.
The new result builds on earlier work by Lukin’s group, using single-atom impurities in diamonds as qubits. Lukin and colleagues have previously shown that these impurities can be controlled by focusing laser light on a diamond lattice flaw, where nitrogen replaces an atom of carbon. That work showed that the so-called “spin degrees of freedom” of these impurities make excellent quantum memory.
Lukin and his co-authors now say that these impurities are also remarkable because, when excited with a sequence of finely tuned microwave and laser pulses, they can emit photons one at a time, so that photons are entangled with quantum memory. Such a stream of photons can be used for secure transmission of information.
Lukin and Togan’s co-authors on the Nature paper are Yiwen Chu, Alexei Trifonov, Jeronimo Maze, and Alexander S. Zibrov, all at Harvard; Liang Jiang of Harvard and the California Institute of Technology; Lilian I. Childress of Harvard and Bates College; M.V. Gurudev Dutt of Harvard and the University of Pittsburgh; A.S. Sorensen at the University of Copenhagen; and Philip R. Hemmer of Texas A&M University. The work was supported by the Defense Advanced Research Projects Agency, the Harvard-MIT Center for Ultracold Atoms, the National Science Foundation, the National Defense Science & Engineering Graduate Fellowship, and the Packard Foundation.