Looking to supercold atoms for answers
Supercooling atoms changes their behavior
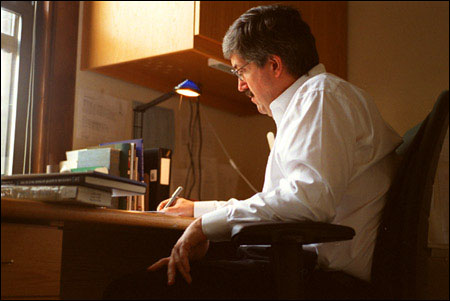
Atoms do weird things when they crowd together and get very cold.
For one thing, they can act like waves instead of the round, little particles pictured in popular science articles and on television shows. When this happens, strange states of matter occur, like fluids that flow without friction and clouds of atoms that interfere with each other like waves in a pond.
Physicists love to experiment with frigid, wavelike atoms because they may reveal unknown things about the beginning of the universe and why it is like it is today. Barring that, the research could lead to superfast computers smaller than a pinhead.
Until a few months ago, the potential of using supercold atoms to answer some of the most basic questions about matter and the universe was limited by the kind of atoms that could be used in these experiments. It was thought that only a few types of atoms could be herded together so closely they behaved more like a slinky than a clump of tiny particles.
But new experiments conducted by John Doyle and his colleagues at Harvard University show that, if you do things right, you can get many different atoms to morph into a so-called Bose-Einstein condensate.
“Until we did this work, no one thought it was possible,” says Doyle, a professor of physics. “Now we should be able to make Bose-Einstein condensates with a wider variety of atoms than ever done before, probably more than half of the hundred or so types of atoms in existence.”
Examining certain cold atoms should give us a new window on how the universe was born, Doyle believes. Besides the matter making up all persons, places, and things we are familiar with, antimatter exists in the universe. The two states are so antagonistic that when they meet they annihilate each other in a burst of energy. All theories about the beginning of everything agree that matter and antimatter must have been originally created in equal amounts. If that’s so, what happened to antimatter? How come we and our houses, food, and electronics are not made of antimatter?
Answering such questions also bears on all the physical standards we think of as constant, like the speed of light. “Finding out that these sacrosanct numbers have changed during the 15 billion year history of the universe would be a dramatic discovery,” Doyle says. “It would change all our ideas about the history of the universe.”
Superbehavior
The idea that atoms could get into a wavy state was first proposed by Albert Einstein and Indian physicist Satyendra Nath Bose in 1926. Technology had to catch up with theory before such a cold atomic soup could actually be made in a laboratory.
The recipe for a Bose-Einstein condensate requires a refrigerator that can cool atoms to temperatures near minus 460 degrees Fahrenheit, a temperature that slows the movement of atoms to almost nil. All the air has been pumped out of the fridge and the atoms are held in place inside by a strong magnetic field. Under such constraints, atoms do not fly around like bouncing balls and collide with each other as they do at familiar temperatures. Instead, they clump together and they start to behave like waves. If you have the right equipment, you can actually take a picture of these atomic waves.
Doyle compares these waves to the movement of a slinky or a wave generated by holding a rope while you spin it. “When such waves interfere with each other, they create patterns like the ripples produced by pebbles dropped into a quiet pond,” Doyle explains. “The atoms behave very differently than they do as a solid, gas, or liquid. Lacking every-direction bouncing and colliding, the atoms move, or superflow, without resistance.”
No one has figured out yet how to make money with superfluidity, but chances are someone will, especially now that it can be made using many different kinds of atoms.
Until Doyle and his team came along, physicists believed that Bose-Einstein condensates could only be fashioned from round atoms. Such atoms have their outer shells filled with the maximum number of electrons that that orbit can hold. The majority of atoms contain unfilled outer orbits and have shapes more like footballs.
“On a hunch, we investigated titanium, a silver-gray metal made of nonspherical atoms,” Doyle reports. In theory, the football-shaped atoms should collide and twist around, ruining the chances of making the B-E condensate, which requires that all atoms point in the same direction. That didn’t happen. Instead, the atoms collided in the same way that round atoms do.
The team next tried rare earths, elements widespread in clays and used for making abrasives, glass, and ceramics, among other things. These atoms have various nonspherical shapes. “We studied them closely packed at ultralow temperatures and actually saw the effects of them running into each other,” Doyle notes. “It was astounding! They came together just like spherical atoms.”
Rounding out the atoms
Despite a variety of shapes, atoms on their way to becoming a Bose-Einstein condensate become rounded on the outside. Their outside shells become filled with electrons, creating a round cage or covering. “No matter what the shape inside this cage, they are round on the outside,” Doyle explains. “When they run into each other, it’s the outside that counts.”
The researchers did not actually make any new condensates but they packed atoms close enough, cooled them down enough, and watched them closely enough to be sure it can be done. They describe these experiments in the Sept. 16 issue of the scientific journal Nature.
“We now expect to make B-E condensates with a variety of atoms, and to create phases of matter never seen before,” Doyle comments. “In the process, we may be able to see new signs of how the universe was born and evolved.”
They may also come across some commercial applications. Condensate atoms are highly magnetic because their magnetic poles all line up in the same direction. If you use outside magnetism, it’s possible to reverse the direction of this magnetic lineup. Thus you have the ingredients for a new type of digital switch.
In one position, the switch could represent “0,” in the other it could represent “1.” These are the two letters of the binary alphabet and number system, which can be combined into all the words and numbers in existence. Such atomic-sized switches could replace the relatively bulky transistors that now give computers all the abilities that they have.
Atoms are billions of times smaller than transistors, so you could perform the same functions with a much smaller, faster machine, as well as do things beyond the reach of the best existing computers. Doyle gives the example of creating unbreakable codes that would make everything from financial information to anti-terrorism plans more secure.