Scientists reinvent DNA as factories for synthetic molecules
Imitating nature’s ‘elegant efficiency’
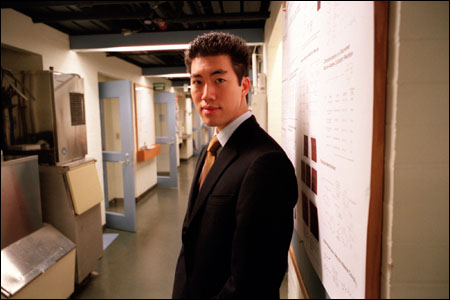
Chemical synthesis is practiced entirely differently by chemists and by cells – and by many measures, cells come out on top. Chemists use something of a brute-force approach, generating vats of superconcentrated chemicals that react through random molecular collisions. Biomolecules are found within cells at concentrations a million times lower, their interactions directed by enzymes that selectively unite sparse biological reactants.
Harvard chemist David R. Liu is trying to inject some of nature’s elegant efficiency into synthetic chemistry, using DNA as an intermediary to introduce synthetic molecules to each other. By piggybacking these small organic molecules onto short strands of DNA, Liu has developed an innovative method of using DNA as a blueprint not for proteins but for complex synthetic molecules.
Liu and his colleagues in the Department of Chemistry and Chemical Biology have published a number of papers on this approach, dubbed “DNA-templated synthesis.” Two recent reports in the journals Science and Nature show that this fundamental method can be applied to diverse problems in the chemical sciences.
“The basic structures of proteins and nucleic acids seem limited when compared with the structures that can be created using modern synthetic chemistry, and yet a very modest set of protein and nucleic acid building blocks has given rise to the incredible complexity and diversity of living systems,” says Liu, the John L. Loeb Associate Professor of the Natural Sciences and associate professor of chemistry and chemical biology in the Faculty of Arts and Sciences. “We’re interested in marrying fundamental features of biomolecules with synthetic organic chemistry.”
DNA-templated synthesis works by attaching organic molecules to single DNA strands, each containing 10 DNA bases (A, C, G, or T). When two DNA strands with complementary sequences (A matches T, G matches C) spontaneously bond together, their associated organic molecules undergo a chemical reaction to generate a product – essentially reinventing DNA as a miniature, programmable assembly line for synthetic chemicals.
Liu’s team has found that small molecules bound to DNA can react to form larger products even when the DNA bases used to zip together the small molecules are far apart on a DNA template. This means that a template strand of 30 DNA bases, complementary to Liu’s DNA codes for three different organic molecules, can encode three separate chemical reactions, leading to the multistep DNA-programmed synthesis of relatively complex cyclic products.
“We recognized that in order to apply such an approach to as many synthetic molecules as possible, we’d have to use a different type of template than an enzyme,” Liu says. “The natural and robust zipping up of complementary DNA strands is a simple way to bring molecules at low concentrations together without having to develop an entirely new class of enzymes for each different type of molecule.”
The 10-base DNA strands used by Liu’s team are large enough to assemble spontaneously at room temperature, and in theory can encode thousands of individual small organic molecules. Because the resulting synthetic compounds remain linked to DNA, techniques long used to screen and amplify the genetic mainstay can be applied. Chemical products can be selected on the basis of ability to bind to a protein target, and the attached DNA code can then be amplified by the polymerase chain reaction (PCR) and sequenced to reveal its identity. A study integrating these developments recently appeared in Science.
In a separate study published last week in Nature, members of Liu’s group reported that DNA-templated synthesis can also be used to mine the chemical universe for new reactions. This approach is so efficient for reaction discovery that a single researcher can evaluate thousands of potential chemical reactions in a two-day experiment. For instance, shortly after they started using DNA-templated synthesis to seek out new chemical reactions, members of Liu’s group discovered an unexpected coupling of two simple hydrocarbons, a terminal alkyne and a terminal alkene, to form a useful and more complex group called a trans-enone.
“A conventional approach to reaction discovery, in which different reaction conditions are examined for their ability to transform one type of substrates into one type of product, may well be the best approach for trying to achieve a specific transformation,” Liu says. “But no one knows what fraction of ‘reactivity space’ has been mined thus far, or even what this space looks like. That’s why we’re intrigued by an approach to reaction discovery that does not focus on any specific combination of substrates but instead can simultaneously examine many combinations.”
Liu’s work is funded by the National Institute of General Medical Sciences at the National Institutes of Health, the Office of Naval Research, the Arnold and Mabel Beckman Foundation, the Searle Scholars Foundation, the Alfred P. Sloan Foundation, and fellowships from Bristol-Myers Squibb and the National Science Foundation.