$15 million grant will study bacteria at work:
They clean up pollution, reduce global warming
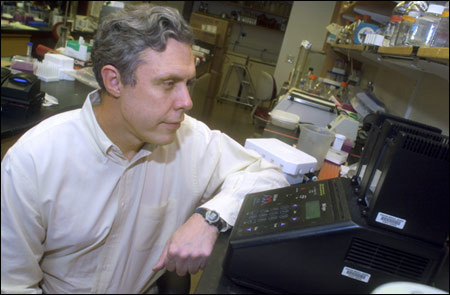
Infectious bacteria that sicken species from worms to humans can be harnessed to clean up chemical spills. Floating microbes, which remove carbon dioxide from ocean air, might reduce the impact of global warming. To explore such capabilities, the Department of Energy (DOE) has awarded $15 million to researchers at the Harvard Medical School, in partnership with the Massachusetts Institute of Technology (M.I.T.) and two Harvard-affiliated hospitals.
The partnership received permission to start using the funds last week (on Oct. 8). The money is part of a $103 million DOE Genomes to Life Program aimed at taking advantage of the natural capabilities of wild bacteria to solve environmental problems.
“It’s very exciting to be part of an interdisciplinary team that will apply the latest techniques of genomics and biology to urgent global issues of energy and ecology,” says George Church, head of the team, professor of genetics at Harvard Medical School, and a faculty member of the Harvard-M.I.T. Division of Health Sciences and Technology.
“The five-year grant will be used to understand the workings of target bacteria so well that we can align human priorities with what they do naturally,” Church adds. “We’re not trying to modify bacteria to make them ‘useful to humans,’” notes Sallie (Penny) Chisholm, professor of engineering and biology at M.I.T. “They already are useful. Sure, we hope to make them more useful, but you have to realize how important they are in the wild state.”
For example, a nautical group of bacteria known as Prochlorococcus removes carbon dioxide from air and fixes it into the carbon content of their own tiny bodies. The more carbon dioxide they take from the air, the less is available to capture the heat that is causing the warm-up of our planet.
That adds up to a lot of carbon. “Prochlorococcus is a major ocean sink for carbon,” Church testifies. “It is responsible for 40 percent of the photosynthesis (carbon dioxide removal) on Earth.” A quart of ocean water often contains 100 million Prochlorococcus cells. Its population in the global ocean could be as high as 10 trillion trillion. The partnership speculates that it “may well be the most abundant organism on this planet.”
Making microbe machines
The $15 million team expects to identify and understand the function of every one of the approximately 1,700 proteins in Prochlorococcus’ microbody. Each protein will be traced back to the gene responsible for producing it. Then Church and his colleagues will build a detailed computer model of how the genes and proteins interact to take carbon dioxide from the air and lock it up in their bodies. Such a working blueprint would show engineers how human activities might affect Prochloococcus’ capabilities.
Millions of years of evolution have engineered Prochlorococcus into a lean, mean photosynthesizing machine. But the details of how it does this still remain mysterious. These bacteria reproduce once a day, but that’s not as impressive as other microbes that replicate every half-hour. Several groups have attempted to fertilize the ocean with iron to increase the reproductive rate of Prochlorosoccus and thus the rate that carbon dioxide is taken out of the air every day.
But experimenters can’t just rain engineered cells down on the ocean without knowing the outcome. Increasing carbon hoarding might increase populations of other microbes that survive by eating the hoarders. (That’s easier than making their own carbon.) But in the course of living and dying, increased hordes of bacteria-eaters could release extra carbon dioxide, defeating the purpose of all the research and engineering of Prochlorococcus cells. By running scenarios in the computer before they try them in the ocean, the Harvard-M.I.T. scientists want to prevent such “oops” situations.
“Adjusting ecological balances, however possible, is not a goal for us,” Church warns. “We provide information and warnings that others will need and heed to carry out those experiments.” He compares this effort to providing computer-aided design and manufacturing guidance to a factory that builds automobiles. “Our team doesn’t produce cars,” he says, “we deliver the deep understanding of how to design and make them.”
A head start
The Harvard-M.I.T partners already have a head start on doing such things. Church’s lab has been studying the genes of microbes since 1987. It developed the technology used for analysis of the human genome and of such harmful bacteria as Helicobacter pylori, the pathogen responsible for ulcers and stomach cancer. Collaborators at M.I.T., led by Penny Chisholm, brought Prochlorococcus from scientific obscurity to its proper place as a heavyweight of carbon-dioxide trapping.
With that kind of background, Church, Chisholm, and their collaborators are a natural fit for cataloging the genes and proteins that DOE wants to exploit. Besides Prochlorococcus, the team will investigate Pseudomonas, a versatile infectious microbe that lives in water, soil, plants, and animals, and Caulobacter, a bacterial group common in freshwater streams. Both can degrade chemical wastes and other pollutants.
Pseudomonas bacteria are well-known for their ability to “eat” their way through aromatic compounds like benzene and naphthalene, as well as toxic and corrosive mixtures of oil and gasoline. With a diet like that, these microbes are toxic themselves. Healthy people can fight off Pseudomonas attacks, but for those whose immune defenses are compromised by disease, such as AIDS, the same infections can be lethal.
“One of our goals, then, might be ways to genetically engineer them to make them safer,” Church points out. “We intend to find out if that’s feasible.”
It’s not going to be easy. Each Pseudomonas contains some 6,000 proteins that make it what it is. That’s more than three and a half times as many proteins as Prochlorococcus has. Not every one reacts with every other one, of course, but interactions of 20 or more may take place simultaneously. Unraveling their interworkings will take time and technology. The partnership is already putting together the instruments needed to identify the proteins and find out which ones are active in different circumstances.
“As we begin the next five years of work, we have specific goals and milestones to reach,” Church says. “However, we can extend or change our goals. That’s one of the gratifying things about science. While pursuing the solution of one problem, you often make unexpected discoveries that lead to more fruitful avenues of research.”