Genes for a better brain found
Discovery surprises neuroscientists
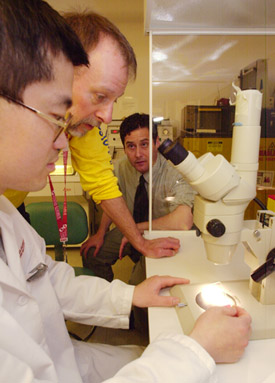
Genes that have allowed brains to become larger and more complicated have been found and cloned by researchers at Harvard Medical School (HMS) and the California Institute of Technology.
One of the genes has been guiding the formation of nervous systems since the first fishlike animals with backbones appeared on Earth. The other evolved about the time that the brains of these vertebrates got larger than those of birds.
In the discovery process, neuroscientists were surprised to learn that their theory about how central nervous systems (brains, spines, and eyes) develop in everything from mice to humans was wrong. This new knowledge has implications for better understanding of human diseases such as multiple sclerosis, mental retardation, and brain cancer. It also heightens the possibility that adult stem cells, present in humans throughout their lives, might someday be used for treatment of these infirmities.
The two genes, known as Olig1 and “Olig2,” contain instructions for making cells (oligodendrocytes) that wrap around nerves in the brain and spine to form an insulating sheath of fat called myelin. Like insulation around copper wires, the myelin allows these nerves to carry messages over relatively long distances, say, from the spine of a tall human to her foot. Backboneless animals (invertebrates) like bugs, worms, and oysters don’t need the covering because their nerve signals have shorter distances to travel.
Olig2 has total responsibility for production of both myelin cells and motor nerves in the spines of mice, humans, and other mammals. “Without Olig2, mice die at birth because they cannot move or breathe,” says Charles Stiles, professor of microbiology and molecular genetics at Harvard-affiliated Dana-Farber Cancer Institute in Boston.
In the brain, both genes cooperate to produce the vital myelin cells.
But this was not known before the work of Stiles and his colleagues. Everyone thought that the major parts of vertebrate brains formed in a different way.
A gene not seen before
Most people are surprised when they learn what kinds of cells make up a human brain. Less than 10 percent of the cells are hard-working neurons responsible for movement, thinking, memory, and other body functions. More than 90 percent are so-called glia cells. That name means “glue” in Latin, because for a long time scientists thought these cells were just the stuff that supports neurons and holds the brain together. Now, scientists know there’s a lot more to it.
Myelin-making oligodendrocytes are one of two types of glial cells. The others, called astrocytes because of their star shape, provide support and nutrition and play a role in establishing the blood-brain barrier that keeps unwanted molecules out of the brain. Even at the present advanced state of knowledge, neuroscientists admit they don’t know everything that astrocytes do. “Their role is multifaceted and somewhat nebulous,” is the way Stiles puts it.
All three types of cells come from stem cells that, during development in the womb and even in adulthood, are capable of forming a number of different cell types. At the top of the hierarchy, one type of stem cell makes daughter cells either for the central nervous system, or for peripheral nerves – those that activate arms, legs, and internal organs.
Stem cells lower down on the central nervous system ladder can make neurons, astrocytes, or myelin cells. Until Stiles and David Rowitch, an assistant professor of pediatrics at HMS, began their experiments two years ago, just about every medical scientist in the world believed that neurons came from one type of stem cell and glial cells came from another.
“It seemed intuitively right,” Stiles notes. “Neurons handle electrical impulses, while glial cells provided support and protection. But we had no direct genetic information to prove such genealogy, and a few voices in the scientific wilderness said that this may not be the way it is.”
To find out for sure, Richard Lu, a postdoctoral fellow in Stiles’ lab, did what Stiles calls “a gutsy experiment.” Lu took stem cells from rat embryos, and with the help of some hormones and growth proteins he coaxed them to grow in a laboratory dish. He then used various genetic techniques to isolate different proteins and genes associated with these stem cells. By this process, he found a gene that had never been seen before, Olig1.
Lu then searched the newly available genomes of humans and other mammals and found a closely related gene, Olig2. Looking at a variety of animal genomes revealed the presence of Olig2 in animals from flies and worms, through birds and fish, to mice and rock stars. Olig1, in contrast was found only in mammals. Stiles guessed that the two genes may once have been one, then they split apart later in evolution.
Tests are a knockout
But the experimenters still didn’t know exactly what these genes do. One way to find out is to eliminate or “knock out” the genes in question, then see what happens. You have to knock out Olig1 in some mice, Olig2 in others, and both genes in yet others. That turns out to be quite difficult. To get it done, Stiles and Rowditch enlisted the help of David Anderson at the California Institute of Technology. Anderson and his team took on the double knockout. Stiles, Rowditch and Lu did the individual KOs.
Without Olig1, mice are born healthy. They suffer a slight delay in myelination but catch up later and go on to breed. Mice without Olig2, or lacking both genes, die at birth because they are completely paralyzed. The missing genes prevent formation of all neurons in the spine necessary for movement and breathing.
The now inescapable conclusion that both neurons and myelin cells come from the same progenitor, while astrocytes have a different parent, was a big surprise. It changes fundamental knowledge on which everything from textbook chapters to medical decisions will be based in the future.
The research results emphatically support the idea that mice and their mammalian relatives possess active cells in their adult brains. Until the 1960s, no one thought it possible to form new brain cells; dogma dictated that you came into the world with a set number of brain cells and that number continued to get smaller and smaller.
“We don’t know the number or potency of stem cells in the adult human brain, but we do know they can form new cells,” Stiles remarks. “This capability provides a possible way around the political and ethical dilemma of using embryonic brain cells to do things like restore movement to paralyzed limbs.”
The new discoveries also have implications for treating multiple sclerosis, an incurable and progressive disease caused by breakdown of myelin in the brain and spinal cord.
The Oligo genes are located on chromosome 21, an extra copy of which is the source of Down syndrome, a birth defect that produces mental retardation and abnormal changes in physical appearance. Some day it may be possible to treat Down syndrome by inhibiting the activity of the extra Oligo genes on the extra chromosome.
The fact that neuroscientists have pinpointed genes vital to formation of the two kinds of glial cells also could be important for treating brain cancers, all of which involve disruptions of these cells.
My lords, we are vertebrate animals, we are mammalia. – W. Maule