New way to “see” DNA
Materials “sculpted” with atomic beams
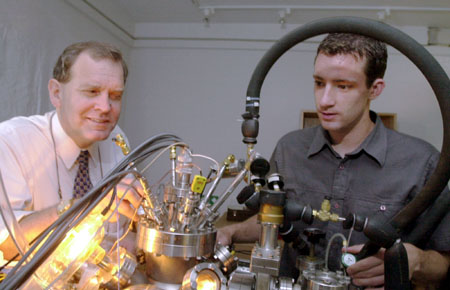
Extraordinarily tiny holes are behind a whole new way to make structures only a few dozen atoms in size.
Such structures may one day make it possible to sequence your genes during a visit to a doctor’s office. A personal gene map, instead of one that generalizes the genes of all humans, would be a first step in determining your risk of heart disease, cancer, and other maladies. That kind of knowledge could help provide a medical strategy for a longer life.
“We have discovered how to move atoms across a surface with such high precision and control that we can make structures to detect and analyze particles as small as a single molecule of DNA,” says Jene Golovchenko, professor of physics and applied physics at Harvard University. DNA molecules carry genes and genes carry the blueprints for proteins and other molecules that control the chemistry of life.
“These devices would be molecular-sized extensions of instruments now used for counting and sorting organic and non-organic particles,” adds Daniel Branton, Higgins Research Professor of Biology. Such particles include contaminants in blood and tiny particles of pollution in air and water.
This research was driven by the need to make extremely small holes that mimic the pores in human cells through which different molecules must pass to keep the cells alive and healthy.
“My colleagues and I first had the idea of sequencing genes by forcing DNA molecules through minute openings,” explains Branton. “We tried to do it with protein pores of the type that channel molecules across cell membranes. But such holes do not remain stable for long periods; heat breaks them down and you can’t attach wires to the sides. Then Jene came up with the idea of making the holes in solid materials.”
Much of the necessary equipment was built from scratch at Harvard’s Department of Physics. “It took us three years,” notes Golovchenko, “and we learned a lot along the way.”
Making small holes smaller
The team began by making the smallest holes possible with commercial equipment. They drilled holes in silicon nitride, a semiconductor, with a beam of electrically charged atoms. The openings were as small as 60 to 100 nanometers in diameter. That’s a scant 60-100 billionths of a meter, hundreds of thousandths of an inch, or some 400 atoms across. Such a hole is too small for even a beam of light to pass through, but it was too big to detect only a single molecule.
Then came a serendipitous discovery. The researchers found that the charged atom beams can move material as well as remove it. Apparently, atoms from the sides of the hole are drawn inward, reducing the hole size The discovery allowed them to use equipment that they custom-built to shrink a 60 nanometer hole until it was only 1.8 nanometers, no wider than 18 atoms.
Details of the feat were published in the July 12 issue of the scientific journal Nature. The report, co-authored by Golovchenko, Branton, Professor of Materials Science Michael Aziz, post-doctoral fellow Jiali Li, and graduate student Derek Stein, is the first to call attention to the possibilities of this new technique known as “ion beam sculpting.”
“This method gives us great control,” notes Derek Stein. “Think of the beam of charged atoms, or ions, as a small stream of water sprayed at a hole in a plate. The smaller the hole the less water gets through. This lets us measure the size of the hole by how many ions pass through. When we reach the right hole size, a computer shuts off the beam.”
The next step involved trying to pull a single strand of DNA though one of the holes, known as nanopores. The researchers made a pore 5 nanometers (about 50 atoms) wide in a silicon nitride membrane suspended in liquid. One side of the liquid held a slight positive charge, the other a slight negative charge. The experimenters added DNA, which carries a slight negative charge, to the negative part of the liquid. That caused it to flow to the positive side.
“It looks like the DNA strand is being sucked through the nanopore like a piece of spaghetti,” Golovchenko says enthusiastically.
More than holes
Future versions of this laboratory device might feature many nanopores working in parallel. DNA strands are built of smaller molecules called bases. Each gene on a strand consists of a long string of four bases, signified by the letters A, C, G and T. These sequences of letters spell out the alphabet of life, what color eyes and hair the bearer will have, what diseases he or she might inherit, what proteins will make that person more or less physically and mentally fit.
The DNA bases each have different sizes and electric properties. As they pass through a nanopore, each one blocks the hole in a different way that can be measured by the resistance to an electric current flowing across the hole.
Government, university, and industrial labs worked for many years, using large facilities and machines that cost hundreds of thousands of dollars each, to determine the sequence of billions of bases that make up 35,000 or so human genes. If things unfold the way Golochenko and Branton hope, relatively inexpensive devices in a doctor’s office should be able to read your genetic blueprints like a ticker tape.
To make such instruments a reality, the Harvard scientists are collaborating with Agilent Technologies Inc. of Palo Alto, Calif. The research is also supported by the Defense Advanced Research Projects Agency.
Also, there’s a lot more going on than just making small holes.
The ability to move atoms around on a surface using controlled atomic beams may have important applications in many other fields including the making of extremely small electronic components for computers, pagers, cell phones, and myriad other devices.
“We also know that ion beam sculpting works on many different kinds of materials and structures,” Golovchenko points out. “I think it will become an important method for anyone who wants to make devices or instruments that work on a molecular scale. It’s really incredible that we have the tools to do that.”