Cell development is reversed
May someday lead to regeneration of heart, other organs
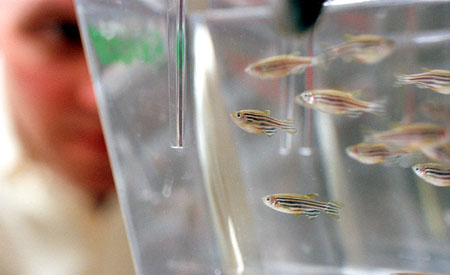
If the lizardy newt loses a leg in a battle with a stronger, faster rival, it simply grows a new limb.
Why can’t humans do that? Mark Keating, a professor of cell biology at Harvard Medical School, asked himself this question about eight years ago. Most people in the field doubted such a thing could be done, but he decided to look into it anyway.
After years of setbacks, Keating has taken a giant step toward understanding the potential for regrowth of limbs and organs. He and his colleagues have managed to manipulate fully developed mouse muscle cells to the point where they revert to stem cells, uncommitted cells capable of growing into any type of cell. Further, he coaxed these cells into starting their lives over again as bone, cartilage, and fat cells.
In other words, he did what everyone thought was impossible. “It’s work that should change the way people think about dedifferentiation (restarting development),” Keating says. Mice, like humans, are mammals; they are much closer to us genetically than newts. Newts are amphibians, more closely related to salamanders and frogs.
Keating and his colleagues published their results in the journal Cell last Dec. 22, but the article didn’t draw much attention. “The journal didn’t do a full-court press to get publicity, and that issue came out when everyone was preoccupied with Christmas holidays,” Keating notes. Nevertheless he considers the work “very important.”
Stem cells are primitive or general cells, present in embryos and capable of developing into heart, brain, eye, muscle, or any other specific cell type. Scientists worldwide are working energetically on ways to use these cells to repair damage done by injury or disease, and even to regrow new organs. Keating sees the possibility of using his technique for reversing degeneration in diseases such as Alzheimer’s and Parkinson’s, stroke and heart disease, and for repairing all types of traumatic injury.
In other words, his breakthrough opens up the possibility, albeit in the distant future, of humans regrowing a leg or arm, much like the long-tailed, slimy-skinned newt.
Scarred hearts
In 1993, Keating was studying a heart problem at the University of Utah when his mind made the turn toward regeneration. His interest centered on the walls of the aorta, which carries blood from the heart to the rest of the body. Damage to this vital vessel activates natural mechanisms that attempt to make repairs. Such repairs, however, result in tough, fibrous patches that are much like scar tissue.
“Thinking more broadly, I came to see this as a common problem,” Keating recalls. “Damage to the brain, muscles and other organs and tissues, or loss of a finger or toe, produce scarring instead of renewal. It is an important field, but one largely ignored because of the firm belief that humans cannot regenerate themselves.”
When a newt loses its leg or tail, a special layer of cells forms over the damaged area, and these cells revert to stem cells. Then they shift into forward gear and specialize into leg or tail cells that effect a complete repair.
Keating began a close study of newts and soon found a gene, called msx-1, which always turns on when the amphibian needs a replacement part. Other researchers had found that msx-1 seems to be associated with the regrowth of the tips of mouse toes lost in traps. Even humans can, under certain conditions, regrow the tip of a finger.
“In both humans and mice, msx-1 is guilty by association,” Keating points out. “There was no proof of a cause-and-effect relationship, but enough circumstantial evidence existed for us to start with this gene.”
After years of what Keating describes as “setbacks and missteps,” he found a way of turning the gene off and on in mouse muscle cells growing in test tubes. When msx-1 was on, other genes stopped producing proteins that make muscle what it is. By adding other molecules, Keating was finally able to push the muscle cells back in development until they became stem cells.
To press these stem cells into developing along different lines, Keating’s team then mixed them with growth factors that enhanced their differentiation into bone, cartilage, and fat cells. The process took only about two weeks.
Could the same thing be done in humans? Could you take skeletal muscle cells grown in lab dishes and turn them into cells that might repair damaged blood vessels and hearts? “Possibly,” Keating answers, “but it’s not something we will know until many more experiments are done.”
Reseeding a trampled brain
Some big problems need to be solved first. Mixing msx-1 with cells in a test tube then turning it on was difficult to accomplish. Getting the same gene into just the right cells in a living mouse or human, and turning it on when needed, will be a much more formidable challenge.
One way is by gene therapy, putting msx-1 into a harmless virus that would infect target cells. It also might be possible to hook the protein produced by msx-1 onto a “tag,” which can bore its way into a cell. Keating admits that he hasn’t had much success with either method.
He is also looking at ways to alter cell development from the outside. A membrane surrounds all cells, but this protective envelope contains protein receptors on its surface. It might be possible to put a protein on such receptors that will trigger the start of dedifferentiation inside those cells.
Also, there may be genes and proteins other than msx-1 that can reverse development. “We’re looking for such molecules,” Keating says.
Recently, it was discovered that certain types of stem cells occur in the brains of young children and even adults. Called neural stem cells, they have the capacity to develop into any cell type the brain needs to work properly. It may someday be possible to use Keating’s technique to shape such cells into replacements for those damaged by a variety of brain disorders from Alzheimer’s disease to stroke.
In the same building where Keating works at Children’s Hospital in Boston, neurologist Evan Snyder works on doing such repairs in mice. “One of my colleagues compares this to reseeding a lawn,” Snyder says. “A birth defect is analogous to not putting down the sod right in the first place. An injury or disease is like a period of bad weather or people trampling over the same spot repeatedly. If you want to start over, you plant new seeds; the seeds for regrowth of the brain are neural stem cells.
Keating wants to make new seeds for all parts of the body. “We work very hard to make things happen as quickly as possible, but no one can build Rome overnight,” he says. “Sometimes we get lucky and things happen faster than we anticipate. More often, the pragmatic application of our work takes a long time to materialize.”