Biological clock genes identified
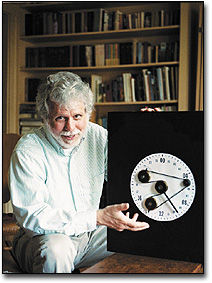
Scientists have gotten the closest look yet at the inner works of biological clocks that drive our natural sleep-wake cycle. Theyre surprised at how complicated the mechanism is.
Steven Reppert of Harvard Medical School and his colleagues have been involved in cloning and characterizing seven genes that operate the natural timepiece deep in the brains of mice and humans. These genes provide blueprints for making proteins that automatically keep our sleep-wake cycle adjusted to the regular coming and going of day and night.
“Weve identified the molecules that we believe form the essential gears of the 24-hour clock,” says Reppert, who is a professor of pediatrics at Harvard-affiliated Massachusetts General Hospital. “This understanding could lead to quicker and more efficient ways to reset the clocks of those suffering from jet lag or genetic sleep disorders, or those trying to adjust to shift work.”
Shift and travel adjustments can be made by exposure to illumination from so-called “light boxes,” but such resetting is time-consuming. As natural sleep potions, Melatonin supplements have not lived up to the hype created by the health food industry.
“My lab cloned the cell receptors through which melatonin exerts its influence,” Reppert notes. “Although it has some effect on the circadian (daily) clock, Im skeptical about its clock-resetting potential.”
Changes in light, brought about by the daily rotation of Earth, wind the biological clocks of every living thing from fruit flies to humans. To provide a predictable structure for our lives, we divide the day evenly into 24-hours. But our internal clocks, as measured by our diurnal tides of hormones and body temperatures, run on a day of 24 hours and 11 minutes. (This period was measured accurately for the first time in 1999 by Charles Czeisler, a Harvard Medical School chronobiologist.)
Left alone, our brain clocks would slow by 11 minutes each day. Evolution, however, has fashioned a clever mechanism of light receptors and clock proteins to reset our internal clocks on a daily basis. When people fly across five or six time zones, say from New York to London or Amsterdam, the clock takes longer to reset. The same is true when workers change from day to night shifts. Also, people with Alzheimers disease and other maladies may have difficulty keeping their biological clocks running on time.
An Explosion of Research
The past four years have seen an explosion of research into the molecular ticking and resetting of inborn clocks. This month, Reppert and colleagues announced the discovery of a previously unknown feedback loop in the clocks of mammals that automatically switches sleep and wakefulness genes on and off as needed.
For ease of experimentation, Repperts mammal studies are done with mice, but he and others believe rodent clocks dont differ much from the human variety. “Clock genes cloned in humans are very similar to those cloned in mice, so we believe that the underlying mechanism is much the same,” he says. “We knock out or eliminate different genes one at a time in mice to determine how that effects clock operation, something thats impractical in humans.”
Morning light starts off the daily cycle when it hits the retina at the back of the eye. Retinal photo cells convert patterns of light to electrical signals that travel via nerves to a small knot of about 10,000 clock cells known as the suprachiasmatic nucleus.
During the day, proteins in each clock cell switch on genes that make other proteins. As night begins, these proteins switch the genes off. The proteins have names like period, timeless, clock, and cryptochrome. The last is also responsible for setting the rhythm of flowering in plants and for sensing light changes that run the daily lives of fruit flies. In humans, the levels of some proteins are rising in the middle of the day; others rise at night.
“We now have a solid understanding of the molecular workings of the biological clocks of mammals,” Reppert comments. “This understanding reveals multiple places where we might be able to use new drugs to quickly reset internal timekeeping. But we still dont know everything. We believe there are other genes and proteins responsible for fine-tuning, and it would be prudent to know how these operate before tinkering with the main gears.”
To find out, Reppert would like to build his own biological clock; that is, take a non-clock cell and put in all the right genes. Once thats done, he and his colleagues have to figure out how to turn these genes on and off, the way nature does it. Once thats been done, they should have enough expertise to guide others in fixing clocks that keep people from getting a good nights sleep every night.
Some people are notoriously early risers; they get up at 4 a.m. and theyre ready to go sleep by 7-8 p.m. Others cant seem to function well during early daylight hours. “You see whole families of such larks or owls,” Reppert notes, “Such odd timing is passed along in their genes, just the way that normal clock genes are.”
A search for lark and owl genes is going on in several other labs. “It will be interesting to see what these researchers find,” Reppert comments. “I predict that it will be some of the same clock genes that were studying.”
Reppert and his colleagues describe their latest findings in the May 12 issue of Science. The cover shows a special sculpture that displays the molecular feedback loops of biological clocks as mechanical pulleys and belts. The sculpture was done by Repperts son, Jason. “It was great fun being able to work together on this,” Reppert says.