With A Song In Their Heads — Birth of new brain cells induced in birds
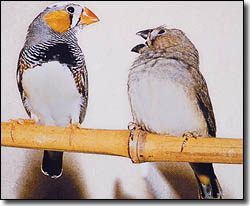
Brain cells that make it possible for zebra finches to sing were forced to die then brought back to life by researchers at Harvard and Rockefeller universities. In a major biological first, quiescent stem cells naturally present in the birds brains were induced to replace the lost cells and restore the finches ability to sing their distinctive song.
Upon the death of mature song cells, immature cells were induced to “awake” from a dormant state. They moved to the right place in the brain, and made connections needed to repair the damage.
“Our results represent the restoration of a brain circuit involved in a complex learned behavior,” says Jeffrey Macklis, associate professor of neurology in the Division of Neuroscience at Harvard Medical School. “It is a step toward doing the same thing in mammals.”
Mammals include humans, and Macklis sees the future possibility of using such approaches to treat damage done by spinal cord injuries, strokes, and degenerative conditions such as Huntingtons and Alzheimers diseases. Even keeping normal brain cells healthy, and thus slowing aging, is not out of the question.
The old ideas that humans lose brain cells as they age, and that these cells cannot be replaced are simply not true. Evidence from experiments with adult monkeys and mice points to the existence of so-called multipotent precursors, immature or uncommitted cells that can develop into different types of brain cells with the proper stimulation.
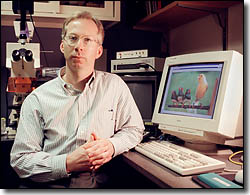
“From direct evidence in the hippocampus of the human brain, it is assumed that human adults also can, to some extent, grow replacement brain cells,” Macklis notes.
During early development, millions of precursor cells are active, but by adulthood most have been used up. However, a small number survive and continue to reproduce. Such potential replacements have been found in at least two places in mammal brains, a part of the hippocampus that deals with learning and memory, and in the olfactory bulb where nerve cells connect the nose to the brain.
“My intuition tells me that conditions do exist under which we can create other types of brain cells,” Macklis says. “The key is learning to understand the molecular controls that govern why certain cell types can or cannot be replaced.”
At the same time, Macklis and his colleagues at Childrens Hospital in Boston work on the feasibility of transplanting immature cells into adult brains to replace cells lost to disease or injury. They have done this successfully in mice.
With a Song in Their Heads
Songbirds are an excellent place to do research on brain restoration; some of them do it naturally every year. Canaries, for example, fall silent each fall when cells in a high-level vocal center of their brains die off. In winter, the cells undergo a wave of rebirth, and canaries are ready to sing again in the spring.
Mammal brains dont have that kind of flexibility, so Macklis and his colleagues, Constance Scharff and Fernando Nottebohm at Rockefeller University in New York City, turned to zebra finches. These songbirds sing a complicated 10- to 12-note song somewhat like that of a canary, but the rate of birth of its song cells is more like that of mammals.
The finches lay down song circuits soon after hatching and dont undergo the dramatic changes that typify canaries. However, their brains do produce a small but constant trickle of new brain cells.
Macklis research team had previously shown that the induced deaths of brain cells in mice can lead to development of the proper type of replacement cells when the brain receives a transplant of multipotent precursors. The scientists asked themselves if the same thing could be done naturally in zebra finches by killing off song cells. In other words, would the population of quiet precursors awake naturally and restore their song without the help of transplants?
The research team used laser light to injure a specific group of song cells in the finches brains. This activity set into action a natural process called apoptosis, whereby abnormal cells commit suicide. The suicide, Macklis theorized, would set off a chain of events that would lead to the replacement of dead cells to death fostering life.
It worked.
There was a direct correlation between the number of old brain cells that died and the number of new brain cells born. At the end of four months, zebra finches who had lost their “voices” began to sing again.
“This is the first specific induction of new brain cells that became successfully integrated into a circuit that controls a complex behavior,” Macklis notes. It follows, by two years, his groups first successful use of transplanted precursors to replace degenerated brain cells in mice.
Overcoming Limitations
The next step was to try to induce the birth of other types of song cells in zebra finch brains. However, the Harvard and Rockefeller experimenters found limitations in what type of replacements they could make. When they attempted to recruit a second cell type, which connects with a different part of the song system, only the first type of cell was formed.
“We think that once precursor cells enter the song system, its easiest for them to respond to signals that make them one kind of brain cell rather than other kinds,” Macklis says. “To get other kinds of replacement cells, were going to have to learn what molecules in the brain control new cell birth and development. Such knowledge should lead to the tailoring of specific types of cells needed to repair damage in specific brain circuits in mammals, such as spinal cord injuries.”
Macklis believes this can be done by solving the mystery of why new cells can flood a canarys brain but are limited to a trickle in zebra finches and mammals. Once such limitations become clear, Macklis believes they can be overcome “by a complex series of control factors able to take immature precursor cells down specific pathways that lead to different types of mature brain cells.” Such cells hold the potential for reversing paralyzing spinal cord injuries or loss of memory in Alzheimers disease and aging.
Some light on how these goals might be accomplished comes from the transplantation of stem cells into the brains of adult mice, and successfully coaxing them into replacing dying cells. Many of the transplanted cells now look and seem to function just like those they replaced.
One key step to success involves transplanting precursors at just the right stage of development. Experiments already done show the most immature cells are not as effective as those that have already made some decisions about what they will become. “We lose some flexibility with this approach, but we gain increased efficiency in getting the different kinds of cells we want,” Macklis explains.
What is learned from this work bears directly on strategies for manipulating precursors already present in the brain. “These cells are tougher to guide down the right paths, but ultimately they offer great power for therapeutic applications,” Macklis says.
“I think our work with birds sets a strong precedent for the idea of manipulating precursors in mammals,” he continues. Of course, the mammal of choice would be humans.
“Some of the cells we targeted in the finch experiments bear a direct relation to those that degenerate in Huntingtons and other nervous system diseases,” Macklis notes. “If we could have a fantasy of where this field could be in another two or three decades, it would include the ability to manipulate precursor cells without the need to transplant them.”
Macklis sees the application of this approach to spinal cord injuries in the next decade. Further away will be attempts to treat diseases such as amyotrophic lateral sclerosis (Lou Gehrigs disease), Huntingtons, and Alzheimers diseases.
“We have much work to do with mice in order to understand the molecular controls and the genes involved,” Macklis points out. “But once thats done, we may also be able to find ways to keep existing brain cells healthier for longer.”
Thats tantamount to delaying aging.